Review Article | Open Access
Progress in the study of toxic effects of drugs on the male reproductive system
Tina Walker1
1Department of Biology, Faculty of Science, Eskisehir Osmangazi University, Eskişehir, Turkey.
Correspondence: Tina Walker (Department of Biology, Faculty of Science, Eskisehir Osmangazi University, Eskişehir, Turkey; E-mail: Tina.G.Walker@hotmail.com).
Asia-Pacific Journal of Pharmacotherapy & Toxicology 2024, 4: 76-84. https://doi.org/10.32948/ajpt.2024.11.01
Received: 20 Oct 2024 | Accepted: 04 Nov 2024 | Published online: 13 Nov 2024
Key words drug toxicity, reproductive toxicity, male infertility, chemotherap
The testes and spermatozoa of the male reproductive system are essential for conception. The testes are vital organs for spermatogenesis and are the primary sites for androgen testosterone release [5]. The quantity, quality, and function of sperm directly determines male fertility, and proper functioning of the testes is crucial for sperm production and maturation. However, as metabolically active organs with a rich blood supply, the testes are highly susceptible to the effects of drugs or chemicals, resulting in impaired spermatogenesis and reduced sperm quality [4]. Thus, reproductive toxicity studies of drugs have become an important part of drug development, pre-market evaluation, and safe management for clinical drug use [6].
Testicular and spermatotoxicity have been reported in varying degrees for many chemotherapeutic agents, immunosuppressants, and antibiotics [7]. Consequently, assessing the effects of these drugs on the male reproductive system and minimizing or avoiding their toxicity in clinical settings has become a significant and challenging research area [8]. Considering the importance of drug-induced testicular and sperm toxicity in both clinical and basic research and the complexity of the related mechanisms, this review aims to systematically summarize and organize the existing research findings. It explores the mechanisms of action of different types of drugs on the male reproductive system and reveals how drugs may adversely affect testicular and sperm functions through direct or indirect pathways. Discussions now frequently include ways to mitigate drug-induced damage to the male reproductive system through drug dose adjustment, treatment regimen optimization, and ancillary protective measures. Through this review, we hope to provide drug toxicity researchers, drug developers, and clinicians with insights into the effects of drugs on male reproductive toxicity and to help them more effectively identify and manage the reproductive toxicity risks of drugs in clinical practice.
Testicular and spermatotoxicity, the central components of male reproductive toxicity, are characterized by direct drug damage to the seminiferous tubules and testicular mesenchymal cells, or indirectly by impaired spermatogenesis through effects on hormone secretion in the hypothalamic-pituitary-gonadal axis. Additionally, drugs may cause DNA damage in testicular tissue cells through oxidative stress mechanisms, which, in turn, affect sperm production and function. Certain medications can even cause sperm DNA fragmentation, lowering sperm quality and increasing the likelihood of genetic abnormalities in progeny or male infertility [13]. Consequently, male reproductive toxicity has gained significant attention in clinical care, especially when young male cancer patients undergo chemotherapy, necessitating consideration of the possible long-term consequences on fertility.
Globally, the issue of male infertility is increasing, with adverse drug reactions being a significant contributing factor. According to the World Health Organization (WHO), approximately 15% to 20% of couples of reproductive age face infertility problems, and testicular dysfunction caused by the toxic effects of drugs accounts for a significant proportion of male infertility cases [14]. Drug-induced reproductive toxicity is an issue that must be carefully considered in young patients, especially in those with future fertility plans. Consequently, the effects of drugs on male reproductive health have not only attracted widespread attention in the medical field but have also become an important ethical consideration in drug development and clinical applications.
In recent decades, advancements in biotechnology and molecular toxicology have led to a better understanding of drug-induced reproductive harm. Numerous preclinical and clinical investigations have demonstrated that some medications can harm the male reproductive system in several ways [15]. These pathways include direct harm to testicular tissue, resulting in spermatogenic cell death and preventing spermatogenic tubules from proliferating and differentiating, or indirect harm to reproductive function by interfering with the hypothalamic-pituitary-gonadal axis and affecting the release of important hormones, such as testosterone [16]. Chemotherapeutic agents such as cyclophosphamide and cisplatin have been widely reported to cause atrophy of the seminiferous tubules, disruption of spermatogenesis, and even permanent sterility [17, 18]. Additionally, several antibiotics, hormonal drugs, antipsychotics, and nonsteroidal anti-inflammatory drugs (NSAIDs) have been shown to have varying degrees of reproductive toxicity [15].
Testicular function
As an important component of the male reproductive system, the testis performs two main functions: spermatogenesis and androgen secretion. The seminiferous tubules in the testis are responsible for spermatogenesis, a process that relies on the synergistic action of various cells including spermatogonia, Sertoli cells, and interstitial Leydig cells [19, 20]. The development of mature spermatozoa results from spermatogonia undergoing multiple cell divisions and differentiations during spermatogenesis. Supporting cells create a blood-testis barrier to shield the spermatogenic environment from harmful elements outside while also providing structural and nutritional support to spermatogenic cells as they mature. Mesenchymal stromal cells, on the other hand, secrete testosterone, a crucial hormone for sustaining spermatogenesis and the development of male sexual traits [21].
The release of testosterone is controlled by the hypothalamic-pituitary-gonadal axis. Studies have shown that the pituitary gland secretes luteinizing hormone (LH), which acts on Leydig cells to stimulate testosterone production. Simultaneously, the hypothalamus secretes gonadotropin-releasing hormone (GnRH), which regulates the secretion of both LH and follicle-stimulating hormone (FSH), thereby controlling the spermatogenesis process [22]. Drugs may disrupt these cells and their roles in various ways, potentially affecting male fertility by impairing spermatogenesis, reducing sperm quality, or causing hormonal imbalances.
Potential mechanisms of drug-induced toxicity
(1) Direct damage. The direct mechanism of drug damage to the testis mainly involves affecting the structure and function of the seminiferous tubules, supporting cells, and spermatogenic cells. According to research reports, many chemotherapeutic drugs (e.g., cisplatin, cyclophosphamide, etc.) have now been found to kill rapidly proliferating cells by affecting the cell cycle, inhibiting DNA replication, and inducing apoptosis [23, 24]. The cytotoxicity of these drugs not only affects cancer cells but also the rapidly dividing spermatogonia, especially the spermatogonia in the seminiferous tubules. Chemotherapeutic drugs usually result in severe shrinkage of the seminiferous tubules and almost complete cessation of spermatogenesis, eventually causing azoospermia or severe oligospermia. This damage may be short-term or permanent, depending on the drug dose and the duration of exposure. When cisplatin is used to treat cancer, studies have revealed that it causes spermatogenic cells in the testicular tissue to undergo apoptosis, impairing spermatogenesis. In particular, cisplatin causes oxidative stress by increasing the generation of reactive oxygen species (ROS), which reduces testicular function and kills spermatogenic cells. This was confirmed by the findings of Ismail, H.Y., et al. who found that cisplatin could damage testicular tissues by decreasing serum testosterone levels, epididymal sperm counts, and oxidants. Additionally, an antioxidant imbalance was detected due to increased malondialdehyde (MDA) and decreased glutathione (GSH) levels in testicular tissues [25]. Hyun-Jung Park discovered that cisplatin had a harmful effect on spermatogenic cells since it drastically decreased the number of spermatogenic cells in the testes of mice treated with it. Furthermore, it repressed the expression of associated genes [26]. Additionally, the alkylating drug cyclophosphamide directly kills spermatogenic cells through its toxic byproducts, causing structural alterations in the spermatogenic tubules and notable suppression of the spermatogenic process [27, 28]. Takeshima found that when cyclophosphamide doses exceeded a certain threshold, the recovery of spermatogenesis was significantly reduced, potentially leading to long-term or permanent infertility [29]. Moreover, certain antibiotics (e.g. as tetracycline and chloramphenicol) have been found to have negative effects on the male reproductive system. These medications may disrupt mitochondrial activity by directly affecting testicular cells, thereby affecting spermatogenesis and sperm quality [25]. According to a study by Tsai, S. J., chronic use of chloramphenicol may cause structural damage to testicular cells, resulting in irreversible spermatogenic problems, and tetracyclines can drastically lower sperm counts and viability [30].
(2) Hormonal interference. Drugs affecting male reproductive system function by interfering with the regulation of the hypothalamic-pituitary-gonadal (HPG) axis are a common indirect mechanism of toxicity. According to the current study by Dwyer et al., drugs can significantly affect the male reproductive system by interfering with the regulation of the hypothalamic-pituitary-gonadal (HPG) axis [31]. The HPG axis is a key endocrine system that regulates the secretion of androgens (e.g., testosterone) and spermatogenesis. Any disruption of this axis results in abnormal testosterone levels, which can affect testicular function and spermatogenesis [32][31]. The hypothalamic-pituitary-gonadal (HPG) axis is the center of human reproduction. This axis includes neuroendocrine networks that integrate a wide range of internal and external inputs to coordinate the reproductive capacity. Gonadotropin-releasing hormone (GnRH) is the primary regulator of reproduction; GnRH controls gonadotropin secretion and subsequently, gonadal (testicular) function [31]. Studies have indicated that steroid hormones (e.g., exogenous testosterone or anabolic steroids) can inhibit the secretion of GnRH and LH (luteinizing hormone) through a negative feedback mechanism, which can lead to a decrease in endogenous testosterone production [33]. Studies by Gu Y. have shown that this inhibitory mechanism is particularly pronounced in exogenous testosterone supplementation or steroid abusers, and is characterized by atrophy of the seminiferous tubules, a significant reduction in sperm count, and possibly even azoospermia [34]. In addition, the regulation of the HPG axis is influenced by a variety of hormones and growth factors. GnRH is the core hormone secreted by the hypothalamus, which controls the secretion of LH and follicle-stimulating hormone (FSH), thereby regulating testosterone and spermatogenesis in general [35]. It has been discovered that any disruption of the HPG axis, including pituitary or hypothalamic dysfunction, can result in insufficient gonadotropin secretion, leading to hypogonadism, which impairs spermatogenesis and lowers male fertility [36]. These studies suggest that during treatment with hormonal drugs or modulation of the HPG axis, the potential effects of these drugs on the male reproductive system should be carefully evaluated. Patients who abuse steroids, especially those who use high doses of hormones, should be alerted to their long-term negative effects on spermatogenic function.
(3) Oxidative stress. Oxidative stress is one of the important mechanisms by which drugs trigger testicular toxicity. Oxidative stress is a pathological process in which reactive oxygen species (ROS) or free radicals are overproduced and exceed the body's antioxidant capacity, thereby triggering cellular damage. The testis is susceptible to oxidative stress because of its active metabolic activity and high level of cell division activity during spermatogenesis [37]. When ROS production of reactive oxygen species exceeds the scavenging capacity of the body's antioxidant system, spermatogenic cells, supporting cells (Sertoli cells), and Leydig cells in the testis are susceptible to damage [38]. According to Akhigbe R, chronic cocaine use leads to testicular cell death and loss of testicular function through the TNF-alpha/nitric oxide/oxidative stress-mediated caspase-dependent apoptotic pathway [38]. Certain drugs (e.g., anticancer drugs, nonsteroidal anti-inflammatory drugs, NSAIDs, antibiotics) can damage testicular cell membranes, proteins, and DNA by increasing the production of ROS [39]. For example, doxorubicin (DOX) is used to treat a variety of cancers; however, DOX-induced oxidative stress can lead to testicular damage [40]. However, according to Malayeri A., they found that Diosmin (DIO) attenuates DOX-induced testicular toxicity through its antioxidant activity [40]. Studies have shown that oxidative stress leads to apoptosis or necrosis of spermatogenic cells, impairs the function of Sertoli and Leydig cells, and destabilizes the microenvironment of the testis [41]. In addition, oxidative stress severely affects spermatozoa viability, motility, and morphology and reduces fertilization, which is strongly associated with male infertility [42]. Studies have shown that oxidative stress-induced sperm DNA fragmentation not only affects sperm fertility, but may also increase the risk of miscarriage and genetic disorders in the offspring.
(4) DNA damage. Drug-induced DNA damage is mainly characterized by damage to the DNA structure of sperm cells and their precursor cells. Drug-induced DNA damage is one of the main mechanisms that affect sperm cells and their precursor cells. Currently, the incidence of tumors remains high worldwide, and chemotherapy drugs are commonly used to treat tumors. These drugs not only target tumor cells but also affect normal cells [43]. Chemotherapy drugs significantly affect the spermatogenesis process by binding directly to DNA, causing cross-linking, breaking, and preventing its replication or repair, ultimately triggering apoptosis. This damage leads to the apoptosis of spermatogenic cells, which prevents spermatogenesis. Alkylating agents such as cisplatin and cyclophosphamide have strong DNA-damaging properties. Cisplatin, a widely used platinum-based chemotherapeutic agent, forms DNA-protein cross-links, induces DNA double-strand breaks, and activates intracellular apoptotic pathways, leading to the death of spermatogonia and other spermatogonial cells [44]. According to a study by Tharmalingam, cisplatin exposure results in a significant reduction in the total number of germ cells in the human fetal testis, with a reduction in the initial loss of spermatogonia, the main lymphoid cells. Additionally, it was shown that cisplatin exposure had a similar effect on the total germ cell count (including spermatogonial stem cells) in prepubertal human testicular tissue [45]. DNA damage not only affects the quantity and quality of sperm but may also profoundly impact the genetic information that sperm passes on to the offspring. Sperm DNA fragmentation is considered an important indicator of male infertility and is associated with decreased sperm motility, reduced fertilization rates, poor embryo development, and an increased risk of miscarriage. Some studies have shown that even when spermatogenesis is restored in men after chemotherapy, the sperm produced may still carry DNA damage, which may adversely affect the health of the offspring. Overall, both chemotherapy and exogenous hormonal agents can interfere with the normal functioning of the male reproductive system by affecting hormone production, triggering oxidative stress, and causing direct cellular damage. Figure 1 shows effects of drugs on the reproductive system.
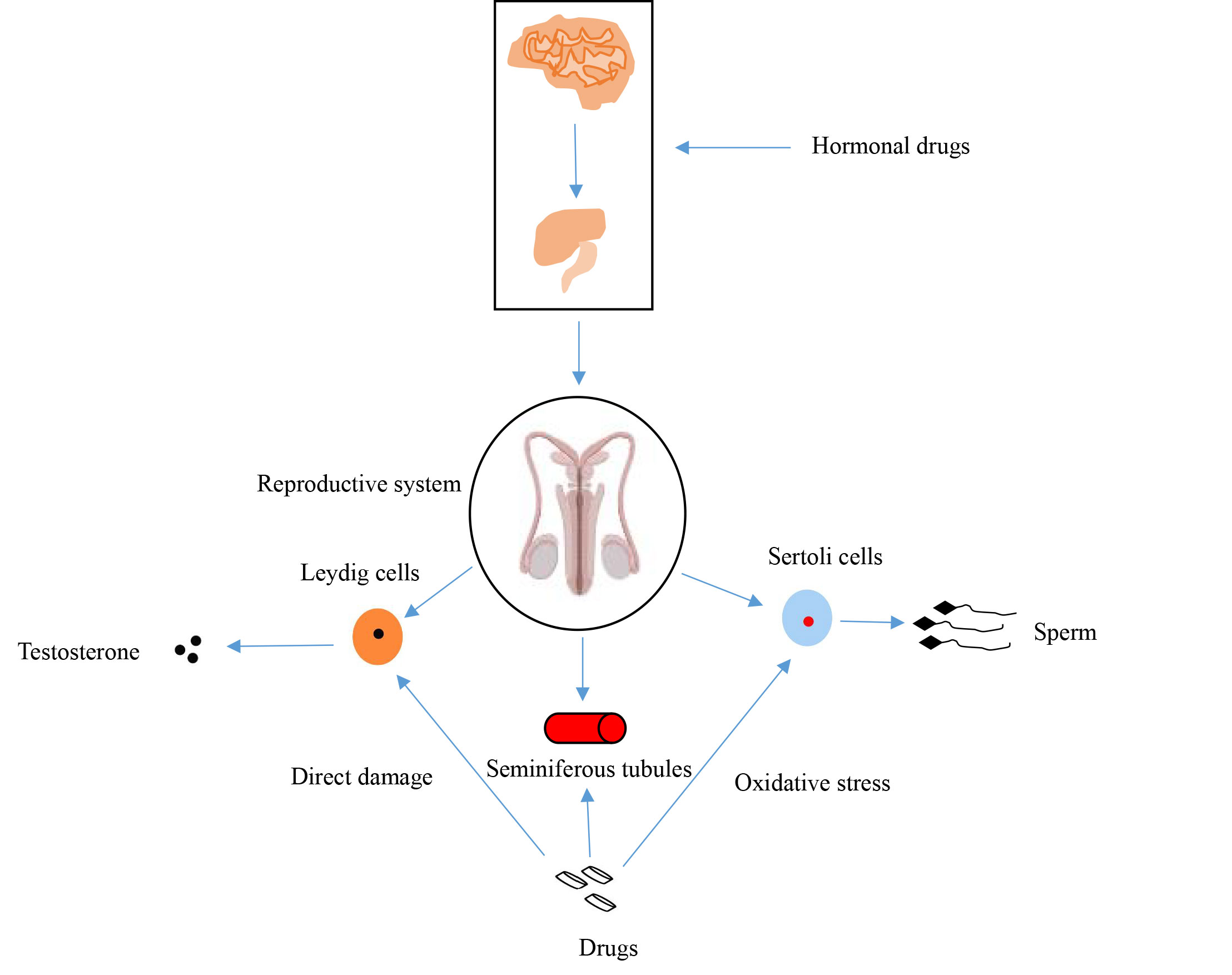
Dose adjustment and treatment optimization
The toxic effects of drugs are usually closely related to the dose and duration of treatment, making dose adjustment and optimization of treatment regimens crucial measures to reduce reproductive toxicity [46]. Different dosages of medication and the determination of the administration duration need to be tailored to different types of diseases. In treating cancer and chronic diseases, which often require prolonged treatment, individualized treatment regimens should be developed based on the patient's reproductive needs, underlying health status, and the risk-benefit ratio of the treatment. For example, certain chemotherapeutic drugs (e.g., cisplatin and cyclophosphamide) are highly toxic to reproduction, and clinical considerations may involve reducing reproductive damage by lowering the dose or shortening the course of treatment.
It has been shown that cisplatin significantly affects spermatogenesis over a range of doses, with long-term effects on the survival of undifferentiated spermatogonial stem cells in particular [47]. Therefore, reducing the dose of cisplatin or employing a phased chemotherapy strategy may decrease the risk of harm to the reproductive system. Similarly, cyclophosphamide dose modifications and real-time dose monitoring have been demonstrated to reduce harmful effects on the reproductive system, especially during long-term treatment [48]. In anticancer therapy, selecting a specific time window for dosing may help to minimize damage to germ cells [47]. For instance, spermatogenic cells are more sensitive to chemotherapeutic agents at specific cell cycle stages; therefore, phasing chemotherapy can minimize interference with spermatogenesis. Additionally, studies have proposed optimizing chemotherapy regimens by modulating the use of cell cycle inhibitors to reduce negative effects on the reproductive system [49]. For some chronic diseases treated over long periods, medication can be appropriately discontinued or intermittently used during the treatment cycle, potentially partially restoring the spermatogenic function.
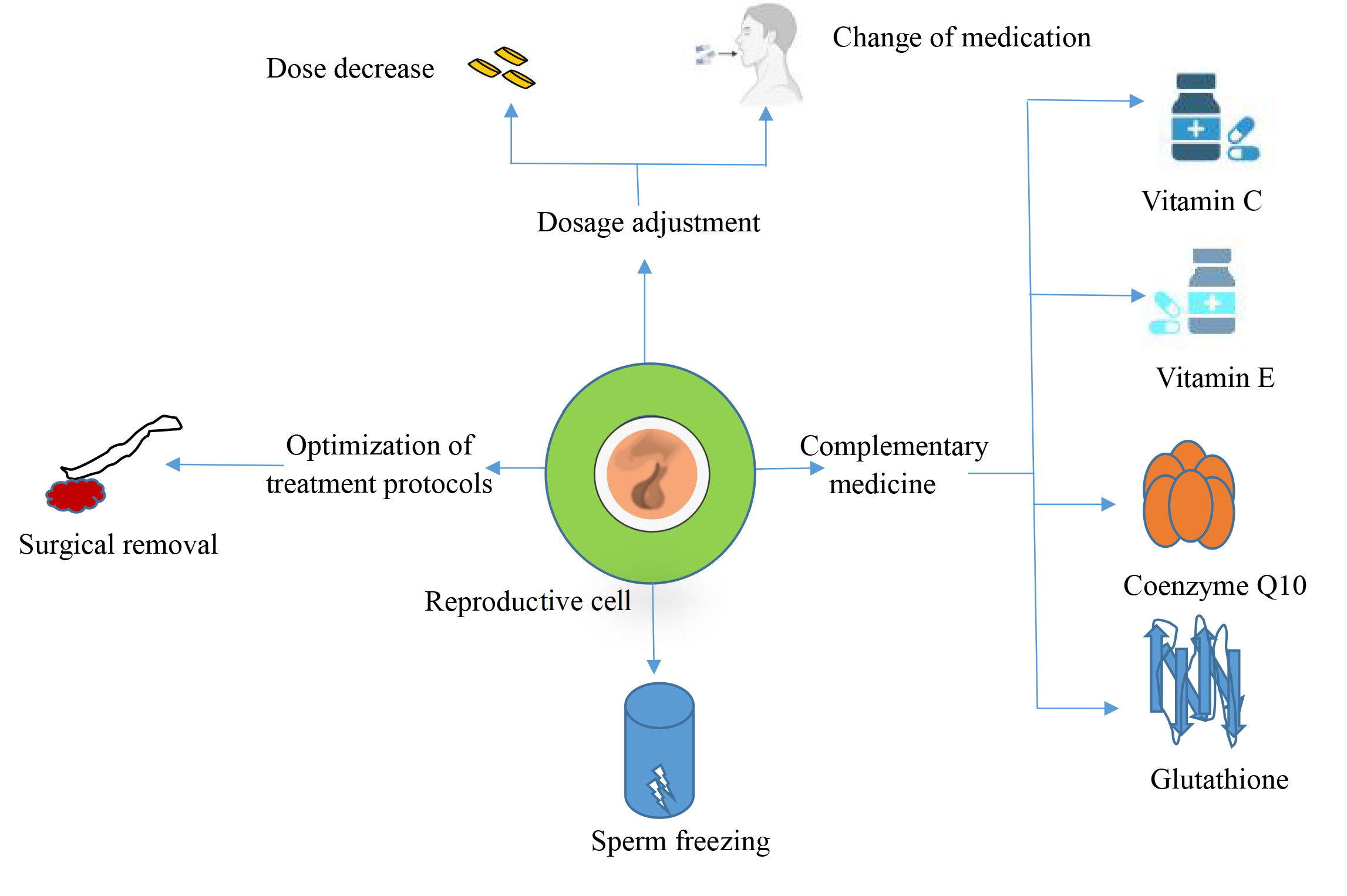
It has been shown that the coenzyme Q10 concentration in seminal plasma is directly related to semen parameters, especially sperm count and motility. CoQ10 concentrations vary in various male infertility states such as varicocele, hypospermia, and pharmacologic or surgical treatments for male infertility, suggesting that CoQ10 plays a vital role in maintaining and improving semen quality [51]. According to Nguyen's study, a micro-supplement containing 60 mg of vitamin E, 400 mcg of folic acid, 30 mg of selenium, 125 mg of L-arginine, 220 mg of L-carnitine, 7.5 mg of coenzyme Q10, 40 mg of L-glutathione, and 20 mg of zinc citrate, supplemented for 3 months, significantly improved sperm quality and decreased the DNA fragmentation index (SDF), thereby reducing oxidative stress-induced DNA damage [52]. This further supports the hypothesis that antioxidants such as glutathione and vitamins C and E can enhance male reproductive health by shielding sperm cells from ROS.
Regarding endogenous hormone suppression caused by exogenous hormones or chemotherapy, hormone replacement therapy can preserve or restore spermatogenic function. Specifically, after discontinuing exogenous testosterone, treatment with a combination of follicle-stimulating hormone (FSH) and human chorionic gonadotropin (HCG) can increase testicular testosterone production and restore spermatogenic function [53]. While FSH promotes sperm production by activating Sertoli cells, HCG functions similarly to luteinizing hormone (LH) by inducing testosterone secretion from Leydig cells [54]. Wenker reported that in a trial of 49 male patients, 95.9% experienced recovery in spermatogenesis following HCG combination therapy, with a mean recovery period of 4.6 months [53]. Furthermore, GnRH analogs have been utilized to increase the pulsatile release of GnRH, which indirectly stimulates spermatogenesis and aids in its restoration by modulating the hypothalamic-pituitary-gonadal axis to enhance endogenous gonadotropin secretion [55].
Methods of resuming treatment
For drugs that produce severe testicular and sperm toxicity, especially hormonal or chemotherapeutic drugs, prompt discontinuation may facilitate the partial or complete recovery of spermatogenic function. Studies have shown that some patients regain spermatogenesis within months to years after drug discontinuation. Recovery depends on the drug type, dosage, length of treatment, and individual patient characteristics. As previously discussed, cisplatin is a common platinum-based chemotherapeutic medication that binds to DNA, forming DNA-protein cross-links and DNA double-strand breaks, significantly impairing rapidly dividing spermatogenic cells [44, 45]. In contrast, recovery after cisplatin chemotherapy shows that approximately 50% of patients regain spermatogenic function after two years, and this percentage can be as high as 80% after five years. The speed and extent of recovery are closely related to the chemotherapy dose and cumulative exposure time [56]. Cyclophosphamide and other chemotherapy drugs can also significantly impact spermatogenesis. The damage caused by cyclophosphamide to spermatogenic cells was dose-dependent, with greater dosages resulting in more pronounced spermatogenic impairment. High-dose exposures may cause long-term decreased fertility, but some individuals may see a return of spermatogenic function within 12 to 24 months of discontinuing the medication. Similarly, spermatogenic activity in patients taking exogenous hormones often returns to normal after three–12 months of discontinuing the medication, although some patients may require more time [57].
Application of reproductive protection measures
Sperm cryopreservation is a crucial fertility protection technique for male patients who require extremely hazardous pharmacological treatments, such as cancer patients receiving chemotherapy or radiation therapy. According to previous studies, spermatogenic activity might be severely impaired and perhaps permanently infertile as a result of cancer therapies, such as chemotherapy and radiation. According to Q Li's study, sperm cryopreservation is now a clinically mature technique that can effectively preserve fertility in patients [58]. Therefore, prior to treatment, patients may choose to have their semen samples cryopreserved for future use in assisted reproductive technologies (ART), such as in vitro fertilization (IVF) or artificial insemination (IUI) to provide reproductive opportunities [59]. Significant variations between sperm quality and post-cryopreservation survival rates were found in this study, which examined the impact of sperm cryopreservation in patients with a range of cancer types, including testicular tumors and hematologic malignancies. For instance, patients with hematologic malignancies had a reduced recovery rate following cryopreservation, but individuals with testicular cancer demonstrated a considerable decline in semen quality both before and after cryopreservation, with a failure probability of almost 10%, whereas frozen sperm conception rates were approximately 28%, miscarriages were 13%, and live births were 20%, according to a systematic review and meta-analysis that included data from over 32,000 patients with cancer [58]. These data further support the importance of fertility preservation prior to cancer treatment and suggest that ART with frozen sperm during or after treatment can be effective in improving fertility chances.
Testicular tissue cryopreservation is an alternative treatment for boys who have not yet reached puberty or for patients with azoospermia. Through cryopreservation of testicular tissue, it is hoped that in the future, techniques such as in vitro culture of spermatogonial cells or testicular transplantation will help patients regain their reproductive function [60, 61]. Although TTC is still in the experimental stage, several studies have demonstrated the potential for generating spermatozoa by testicular tissue transplantation or in vitro culture in animal models. For instance, autologous transplantation has been used to successfully produce viable spermatozoa in primates [61]. Although its practical use in humans has not yet received widespread validation, it might eventually prove to be a useful tool for protecting young cancer patients' fertility. In addition, different response protocols can be designated by drug class for the effects of different drugs on the reproductive system. For example, antibiotics, immunosuppressants, and non-steroidal anti-inflammatory drugs (NSAIDs) [62-64], whether their mechanisms of action are the same and whether the same prevention programs can be used (Table 1).
Table 1. Preventive measures for different drug toxicities. |
||||
Drug type |
Mechanisms of toxicity |
Protective measure |
Complementary medicine/treatment |
Reference |
Chemotherapeutic agent |
DNA damage, oxidative stress |
Antioxidant use, dose adjustment, staged therapy |
Vitamin C, Vitamin E, Coenzyme Q10 |
[43, 44], [50] |
Hormone drugs |
Endocrine regulation disruption |
Discontinuation, hormone replacement therapy |
HCG, FSH combination therapy |
[62] |
Antibiotics |
Mitochondrial dysfunction, direct toxicity |
Dose adjustment, discontinuation |
Coenzyme Q10, Glutathione |
[62, 63] |
Immunosuppressant |
Immunomodulation, cytotoxicity |
Dose monitoring, immunosuppressant replacement |
Methotrexate, Rituximab |
[62] |
Nonsteroidal anti-inflammatory drug (nsaid) |
Oxidative stress, mitochondrial dysfunction |
Antioxidant use, reduced frequency of drug use |
Coenzyme Q10, Coenzyme A |
[64] |
In the future, further development of precise reproductive toxicity assessment methods and individualized treatment protocols will help to better protect male fertility. Meanwhile, the optimization of adjuvant therapy and fertility preservation techniques, such as antioxidant application and cryopreservation of sperm and testicular tissues, should also be the focus of future research. Multidisciplinary collaboration will provide new solutions to minimize drug toxicity and protect patient fertility, especially during the use of high-risk drugs such as cancer therapy, where balancing therapeutic efficacy and fertility protection will become an important clinical challenge. A greater balance between treating illness and reproductive health is anticipated in the future by bolstering ethical considerations in clinical practice and medication development.
No applicable.
Ethics approval
No applicable.
Data availability
The data will be available upon request.
Funding
None.
Authors’ contribution
TW contributed to draft, critical revision of the article and submitted the final manuscript.
Competing interests
None.
- Singh S, Loke YK: Drug safety assessment in clinical trials: methodological challenges and opportunities. Trials 2012, 13: 138.
- Abhishek M, Rubal S, Rupa J, Bikash M: Toxicology of Pharmaceutical Products During Drug Development. Biomed Applica Toxic Nanomat 2023: 187-202.
- Sahota T, Danhof M, Della Pasqua O: Pharmacology-based toxicity assessment: towards quantitative risk prediction in humans. Mutagenesis 2016, 31(3): 359-374.
- Sree BK, Kumar N, Singh S: Reproductive toxicity perspectives of nanoparticles: an update. Toxicology Research 2024, 13(3): tfae077.
- Lopes F, Tholeti P, Adiga SK, Anderson RA, Mitchell RT, Spears N: Chemotherapy induced damage to spermatogonial stem cells in prepubertal mouse in vitro impairs long-term spermatogenesis. Toxicol Rep 2021, 8: 114-123.
- Hoberman AM, Tucker DK: Reproductive Toxicology. In: Drug Discovery and Evaluation: Safety and Pharmacokinetic Assays. Edited by Hock FJ, Pugsley MK. Cham: Springer International Publishing, 2022: 1-19.
- Eid AH, Abdelkader NF, Abd El-Raouf OM, Fawzy HM, El-Denshary E-E-DS: Carvedilol alleviates testicular and spermatological damage induced by cisplatin in rats via modulation of oxidative stress and inflammation. Arch Pharm Res 2016, 39(12): 1693-1702.
- Zhang J, Campion S, Catlin N, Reagan WJ, Palyada K, Ramaiah SK, Ramanathan R: Circulating microRNAs as promising testicular translatable safety biomarkers: current state and future perspectives. Arch Toxikol 2023, 97(4): 947-961.
- Khushboo M, Murthy MK, Devi MS, Sanjeev S, Ibrahim KS, Kumar NS, Roy VK, Gurusubramanian G: Testicular toxicity and sperm quality following copper exposure in Wistar albino rats: ameliorative potentials of L-carnitine. Environ Sci Pollut Res Int 2018, 25(2): 1837-1862.
- Sharma K, Sharma A, Bhatnagar P: Combined effect of polystyrene nanoplastic and di-n-butyl phthalate on testicular health of male Swiss albino mice: analysis of sperm-related parameters and potential toxic effects. Environmental Science and Pollution Research 2024, 31(16): 23680-23696.
- Ginsberg JP: Gonadotoxicity of Cancer Therapies in Pediatric and Reproductive-Age Males. In: Oncofertility Medical Practice: Clinical Issues and Implementation. Edited by Gracia C, Woodruff TK. New York, NY: Springer New York, 2012: 15-23.
- Abdel-Latif R, Fathy M, Anwar HA, Naseem M, Dandekar T, Othman EM: Cisplatin-Induced Reproductive Toxicity and Oxidative Stress: Ameliorative Effect of Kinetin. Antioxidants 2022, 11(5): 863.
- Bry-Gauillard H, Belin F, Vinolas C, Renoult-Pierre P, Massin N, Young J, Sifer C, Grynberg M: Live birth after in-vitro maturation of oocytes in a patient with specific ovarian insufficiency caused by long-term mitotane treatment for adrenocortical carcinoma. Reprod Biomed Online 2022, 44(2): 304-309.
- Anawalt BD: Approach to Male Infertility and Induction of Spermatogenesis. The Journal of Clinical Endocrinology & Metabolism 2013, 98(9): 3532-3542.
- Hamed MA, Ekundina VO, Akhigbe RE: Psychoactive drugs and male fertility: impacts and mechanisms. Reprod Biol Endocrinol 2023, 21(1): 69.
- Rachamalla M, Chinthada J, Kushwaha S, Putnala SK, Sahu C, Jena G, Niyogi S: Contemporary Comprehensive Review on Arsenic-Induced Male Reproductive Toxicity and Mechanisms of Phytonutrient Intervention. Toxics 2022, 10(12): 744.
- Rabaça A, Sousa M, Alves MG, Oliveira PF, Sá R: Novel Drug Therapies for Fertility Preservation in Men Undergoing Chemotherapy: Clinical Relevance of Protector Agents. Curr Med Chem 2015, 22(29): 3347-3369.
- Ghafouri-Fard S, Shoorei H, Abak A, Seify M, Mohaqiq M, Keshmir F, Taheri M, Ayatollahi SA: Effects of chemotherapeutic agents on male germ cells and possible ameliorating impact of antioxidants. Biomed Pharmacother 2021, 142: 112040.
- Wistuba J, Neuhaus N, Nieschlag E: Physiology of Testicular Function. In: Andrology: Male Reproductive Health and Dysfunction. Edited by Nieschlag E, Behre HM, Kliesch S, Nieschlag S. Cham: Springer International Publishing 2023: 15-54.
- Shah W, Khan R, Shah B, Khan A, Dil S, Liu W, Wen J, Jiang X: The Molecular Mechanism of Sex Hormones on Sertoli Cell Development and Proliferation. Front Endocrinol (Lausanne) 2021, 12: 648141.
- Corradi PF, Corradi RB, Greene LW: Physiology of the Hypothalamic Pituitary Gonadal Axis in the Male. Urol Clin North Am 2016, 43(2): 151-162.
- Chu X, Javed A, Ashraf MF, Gao X, Jiang S: Primary culture and endocrine functional analysis of Leydig cells in ducks (Anas platyrhynchos). Front Endocrinol (Lausanne) 2023, 14: 1195618.
- Jahan S, Munawar A, Razak S, Anam S, Ain QU, Ullah H, Afsar T, Abulmeaty M, Almajwal A: Ameliorative effects of rutin against cisplatin-induced reproductive toxicity in male rats. BMC Urol 2018, 18(1): 107.
- Ahmed HA, Gatea FK, Hussein ZA: Azilsartan as a preventive agent against cyclophosphamide-induced testicular injury in male rats. Naunyn Schmiedebergs Arch Pharmacol 2024, Epub ahead of print.
- Ismail HY, Shaker NA, Hussein S, Tohamy A, Fathi M, Rizk H, Wally YR: Cisplatin-induced azoospermia and testicular damage ameliorated by adipose-derived mesenchymal stem cells. Biological Research 2023, 56(1): 2.
- Park H-J, Kim J-S, Lee R, Song H: Cisplatin Induces Apoptosis in Mouse Neonatal Testes Organ Culture. Int J Mol Sci 2022, 23(21): 13360.
- Hiraga H, Ozaki T: Adjuvant and neoadjuvant chemotherapy for osteosarcoma: JCOG Bone and Soft Tissue Tumor Study Group. Jpn J Clin Oncol 2021, 51(10): 1493-1497.
- Ledingham D, Plant M, Mustafa F, Patil B: Preserving fertility: using cyclophosphamide and other cytotoxics in young people. Pract Neurol 2020, 20(2):148-153.
- Takeshima T, Mimura N, Aoki S, Saito T, Karibe J, Usui K, Kuroda S, Komeya M, Yumura Y: Pre- and post-chemotherapy spermatogenesis in male patients with malignant bone and soft tissue tumors. Front Pharmacol 2023, 14: 1324339.
- Tsai S-J, Li L-H, Chen W-J, Huang EY-H, Huang C-Y, Brannigan RE, Huang WJ, Huang IS: Prediction of microdissection testicular sperm extraction outcomes of azoospermic patients post-chemotherapy using cyclophosphamide equivalent dose. Journal of Assisted Reproduction and Genetics 2023, 40(8): 2013-2020.
- Dwyer AA, Quinton R: Anatomy and Physiology of the Hypothalamic-Pituitary-Gonadal (HPG) Axis. In: Advanced Practice in Endocrinology Nursing. Edited by Llahana S, Follin C, Yedinak C, Grossman A. Cham: Springer International Publishing 2019: 839-852.
- Singh V, Agrawal NK, Verma R, Singh K: HPG Axis: The Central Regulator of Spermatogenesis and Male Fertility. In: Male Infertility: Understanding, Causes and Treatment. Edited by Singh R, Singh K. Singapore: Springer Singapore 2017: 25-36.
- Westfield G, Kaiser UB, Lamb DJ, Ramasamy R: Short-Acting Testosterone: More Physiologic? Front Endocrinol (Lausanne) 2020, 11: 572465.
- Gu Y, Liang X, Wu W, Liu M, Song S, Cheng L, Bo L, Xiong C, Wang X, Liu X et al: Multicenter contraceptive efficacy trial of injectable testosterone undecanoate in Chinese men. J Clin Endocrinol Metab 2009, 94(6): 1910-1915.
- Oduwole OO, Peltoketo H, Huhtaniemi IT: Role of Follicle-Stimulating Hormone in Spermatogenesis. Front Endocrinol (Lausanne) 2018, 9: 763.
- Hackney AC: Hypogonadism in Exercising Males: Dysfunction or Adaptive-Regulatory Adjustment? Front Endocrinol (Lausanne) 2020, 11: 11.
- Dutta S, Sengupta P, Slama P, Roychoudhury S: Oxidative Stress, Testicular Inflammatory Pathways, and Male Reproduction. Int J Mol Sci 2021, 22(18): 10043.
- Akhigbe R, Ajayi A: Testicular toxicity following chronic codeine administration is via oxidative DNA damage and up-regulation of NO/TNF-α and caspase 3 activities. PLoS One 2020, 15(3): e0224052.
- Mani S, Tyagi S, Pal KV, Jaiswal H, Jain A, Gulati A, Singh M: Drug-Induced Oxidative Stress and Cellular Toxicity. In: Free Radical Biology and Environmental Toxicity. Edited by Kesari KK, Jha NK. Cham: Springer International Publishing; 2021: 73-113.
- Malayeri A, Birgani SM, Basir Z, Kalantar H: Protective effects of diosmin on doxorubicin-induced testicular toxicity in rat. Naunyn-Schmiedeberg's Archives of Pharmacology 2024, 397(10): 7881-7890.
- Darbandi M, Darbandi S, Agarwal A, Sengupta P, Durairajanayagam D, Henkel R, Sadeghi MR: Reactive oxygen species and male reproductive hormones. Reproductive Biology and Endocrinology 2018, 16(1): 87.
- Agarwal A, Sengupta P: Oxidative Stress and Its Association with Male Infertility. In: Male Infertility: Contemporary Clinical Approaches, Andrology, ART and Antioxidants. Edited by Parekattil SJ, Esteves SC, Agarwal A. Cham: Springer International Publishing; 2020: 57-68.
- Jin Z, Zhao-Xia L, Fan-Ke P, Wen-Juan Z, Min-Li W, Han-Yi Z: Progress in the study of reproductive toxicity of platinum-based antitumor drugs and their means of prevention. Front Pharmacol 2024, 15: 1327502.
- Zhou J, Kang Y, Chen L, Wang H, Liu J, Zeng S, Yu L: The Drug-Resistance Mechanisms of Five Platinum-Based Antitumor Agents. Front Pharmacol 2020, 11: 343.
- Tharmalingam MD, Matilionyte G, Wallace WHB, Stukenborg J-B, Jahnukainen K, Oliver E, Goriely A, Lane S, Guo J, Cairns B et al: Cisplatin and carboplatin result in similar gonadotoxicity in immature human testis with implications for fertility preservation in childhood cancer. BMC Medicine 2020, 18(1): 374.
- Brock K, Homer V, Soul G, Potter C, Chiuzan C, Lee S: Is more better? An analysis of toxicity and response outcomes from dose-finding clinical trials in cancer. BMC Cancer 2021, 21(1): 777.
- Delessard M, Saulnier J, Rives A, Dumont L, Rondanino C, Rives N: Exposure to Chemotherapy During Childhood or Adulthood and Consequences on Spermatogenesis and Male Fertility. International Journal of Molecular Sciences 2020, 21(4): 1454.
- Smart E, Lopes F, Rice S, Nagy B, Anderson RA, Mitchell RT, Spears N: Chemotherapy drugs cyclophosphamide, cisplatin and doxorubicin induce germ cell loss in an in vitro model of the prepubertal testis. Sci Rep 2018, 8(1): 1773.
- Mills CC, Kolb EA, Sampson VB: Development of Chemotherapy with Cell-Cycle Inhibitors for Adult and Pediatric Cancer Therapy. Cancer Res 2018, 78(2): 320-325.
- Kaltsas A: Oxidative Stress and Male Infertility: The Protective Role of Antioxidants. Medicina 2023, 59(10): 1769.
- Alahmar AT, Calogero AE, Singh R, Cannarella R, Sengupta P, Dutta S: Coenzyme Q10, oxidative stress, and male infertility: A review. Clin Exp Reprod Med 2021, 48(2): 97-104.
- Nguyen ND, Le MT, Tran NQT, Nguyen QHV, Cao TN: Micronutrient supplements as antioxidants in improving sperm quality and reducing DNA fragmentation. Basic and Clinical Andrology 2023, 33(1): 23.
- Wenker EP, Dupree JM, Langille GM, Kovac J, Ramasamy R, Lamb D, Mills JN, Lipshultz LI: The Use of HCG-Based Combination Therapy for Recovery of Spermatogenesis after Testosterone Use. The Journal of Sexual Medicine 2015, 12(6): 1334-1337.
- Hakky T, Shirazi H, Christensen M, Pastuszak A: 5 Continuous Testosterone Therapy with Recombinant FSH & HCG Improves Semen Parameters: A Pilot Study. J Sex Med 2022, 19(Supplement_1): S3-S3.
- Isidori AM, Sansone A, Gianfrilli D: Hormonal Treatment of Male Infertility: Gonadotropins and Beyond. In: Endocrinology of the Testis and Male Reproduction. Edited by Simoni M, Huhtaniemi IT. Cham: Springer International Publishing 2017: 1071-1090.
- Howell SJ, Shalet SM: Spermatogenesis After Cancer Treatment: Damage and Recovery. JNCI Monographs 2005, 2005(34): 12-17.
- Mehta A, Sigman M: The Effect of Cancer Therapies on Sperm: Current Guidelines. In: Biennial Review of Infertility: Volume 2, 2011. Edited by Racowsky C, Schlegel PN, Fauser BC, Carrell DT. Boston, MA: Springer US; 2011: 117-131.
- Li Q, Chuan H: P-400 Fertility preservation in male patients with cancer: a systematic review and meta-analysis. Human Reproduction 2023, 38(Supplement_1).
- Liu X, Liu B, Liu S, Xian Y, Zhao W, Zhou B, Xiao X, Wang L, Zhu X, Shu B et al: Male cancer patient sperm cryopreservation for fertility preservation: 10-year monocentric experience. Basic Clin Androl 2021, 31(1): 24.
- Picton HM, Wyns C, Anderson RA, Goossens E, Jahnukainen K, Kliesch S, Mitchell RT, Pennings G, Rives N, Tournaye H et al: A European perspective on testicular tissue cryopreservation for fertility preservation in prepubertal and adolescent boys. Human Reproduction 2015, 30(11): 2463-2475.
- Moussaoui D, Surbone A, Adam C, Diesch-Furlanetto T, Girardin C, Bénard J, Vidal I, Bernard F, Busiah K, Bouthors T et al: Testicular tissue cryopreservation for fertility preservation in prepubertal and adolescent boys: A 6 year experience from a Swiss multi-center network. Front Pediatr 2022, 10: 909000.
- Cho H-W, Lee S, Min K-J, Hong JH, Song JY, Lee JK, Lee NW, Kim T: Advances in the Treatment and Prevention of Chemotherapy-Induced Ovarian Toxicity. Int J Mol Sci 2020, 21(20): 7792.
- Haghi-Aminjan H, Asghari MH, Farhood B, Rahimifard M, Hashemi Goradel N, Abdollahi M: The role of melatonin on chemotherapy-induced reproductive toxicity. J Pharm Pharmacol 2017, 70(3): 291-306.
- Tian En L, Brougham MFH, Wallace WHB, Mitchell RT: Impacts of platinum-based chemotherapy on subsequent testicular function and fertility in boys with cancer. Hum Reprod Update 2020, 26(6): 874-885.
- Mozdzanowska K, Maiese K, Furchner M, Gerhard W: Treatment of influenza virus-infected SCID mice with nonneutralizing antibodies specific for the transmembrane proteins matrix 2 and neuraminidase reduces the pulmonary virus titer but fails to clear the infection. Virology 1999, 254(1): 138-146.
- Hampson AW, Osterhaus AD, Pervikov Y, Kieny MP: Report of the second meeting on the development of influenza vaccines that induce broad-spectrum and long-lasting immune responses, World Health Organization, Geneva, Switzerland, 6-7 December 2005. Vaccine 2006, 24(23): 4897-4900.
- Zhang J, Huang L, Liao M, Qi W: Comparative efficacy of inactivated and live attenuated influenza vaccines. Lancet Microbe 2023, 4(2): e70-e71.
Asia-Pacific Journal of Pharmacotherapy & Toxicology
p-ISSN: 2788-6840
e-ISSN: 2788-6859
Copyright © Asia Pac J Pharmacother Toxicol. This work is licensed under a Creative Commons Attribution-NonCommercial-No Derivatives 4.0 International (CC BY-NC-ND 4.0) License.