Review Article | Open Access
Recent approaches in clinical trials of hepatitis C virus vaccine, challenges and future directions
Muhammad Shoaib Akhtar1
1Department of Cell Biology and Human Anatomy School of Medicine, University of California Davis, California 95616, USA.
Correspondence: Muhammad Shoaib Akhtar (Department of Cell Biology and Human Anatomy School of Medicine, University of California Davis, California 95616, USA; E-mail: xoaib@gmail.com).
Asia-Pacific Journal of Pharmacotherapy & Toxicology 2024, 4: 55-64. https://doi.org/10.32948/ajpt.2024.09.24
Received: 24 Sep 2024 | Accepted: 30 Sep 2024 | Published online: 09 Oct 2024
Key words animal models, HCV, prophylactic vaccine, immune responses
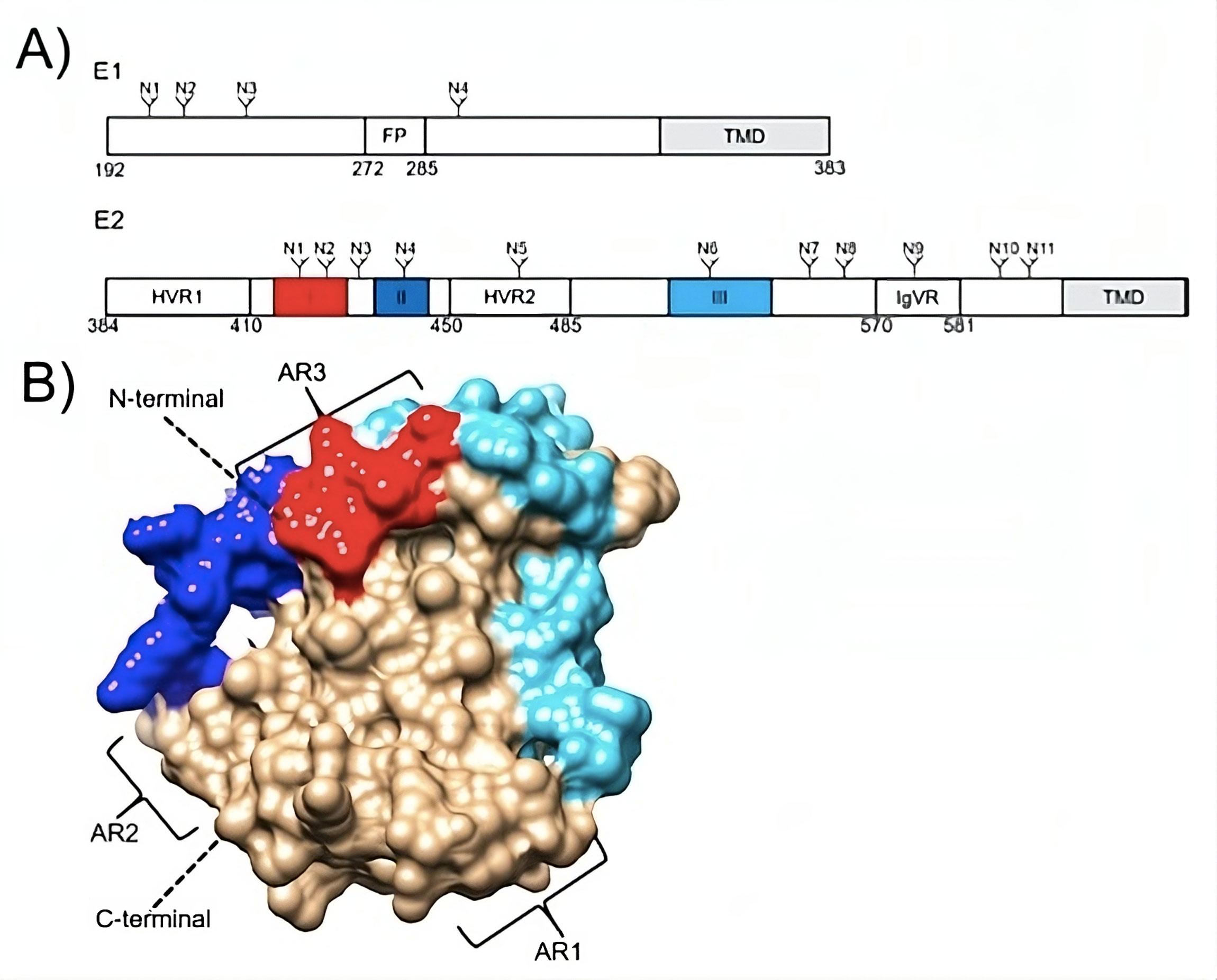
- These vaccines have the potential to deal with the viral genetic diversity and normally they required conserved viral regions as a target.
- The ‘magnitude’ of viral control correlated with antiviral immunity is not exactly explained. However, humoral, broad, functional T cell immune feedback will be essential.
- To be harmless, an effective vaccine will be required to eliminate HCV from the liver without influencing liver immunopathology. Human experiments up till now described that this is a faithful idea.
Table 1. Recent Approaches for the Development of HCV Vaccine. |
|||||||
Vaccine |
HCV strain |
HCV target |
Tested species |
CD8 T cell response |
CD4 T cell response |
Antibody response |
Ref. |
pVax-N3-NS5b |
Gt1b, Gt3a |
NS3, NS4, NS5b |
mice |
N.D |
yes |
homologous, heterogeneous towards Gt1a, 1b, 2a, 2b, 3a, 4a, 5, 6 |
[64] |
DREP- HCV/MVA-HCV |
Gt1aH77 |
core, E1, p7, NS2, NS3 |
mice |
yes |
yes |
non-neutralising IgG |
[65] |
pVax-sE1E2-IMX313P |
Gt1b |
E1, E2 |
mice |
yes |
yes |
N/A |
[66] |
HCVp6-MAP |
Gt4a ED43 |
E1, E2, NS4b, NS5a, NS5b |
mice |
yes |
yes |
homologous, heterologous towards JFH1 |
[67] |
P7 |
Gt1b J4 |
P7 |
mice |
yes |
yes |
N/A |
[68] |
ChAd3/MVA-Nsmut |
Gt1a BK |
NS3, NS4a, NS4b, NS5a, NS5b |
humans |
yes |
yes |
N/A |
[69] |
HBV/HCV-LPs |
Not stated |
linear E1 and E2 epitopes |
mice |
N.D |
N.D |
heterologous towards Gt1a, 1b and 2a |
[70] |
HBV/HCV-LPs |
Gt1a H77 |
E1, E2 |
rabbit |
N.D |
N.D |
homologous, heterologous towards Gt1a and 1b |
[71] |
Core, E1, E2 from Gt1a, 1b, 2a and 3a |
Gt1a H77, Gt1b BK, Gt2a JFH1, Gt3a |
core, E1, E2 |
mice, pigs |
yes |
yes |
homologous neutralising antibodies |
[53] |
H77 sE2Δ123 |
Gt1a H77 |
E2 core |
pigs |
N.D |
N.D |
homologous and heterologous |
[72] |
HCV-1 rE1E2 |
Gt1a HCV-1 |
E1, E2 |
humans |
yes |
yes |
homologous and heterologous |
[72] |
N.D (not determined) and N/A (not applicable). |
Recombinant protein use as a potential vaccination approach may consider that enhancing the immune response to fewer viral epitopes is an appropriate way to develop defensive immunity. Finding the gene encoding the necessary protein and cloning it into yeast, bacteria, or mammalian cells is the major objective of this approach. It is possible to create recombinant proteins using culture material or transfected cells. However, certain recombinant proteins are readily available alone, whilst others need adjuvant therapy to possibly elicit an immune response. Protein-based strategies generally elicit CD4+ T-cell and antibody responses.
Core proteins
As a therapeutic vaccine approach (GI5005) heat-killed yeast cells (Saccharomyces cervisiae) have experimented which showed core-NS3 fusion protein [39]. During Phase II placebo-controlled experiment in 66 chronic HCV-1 patients, GI5005 was integrated with excellent therapy (PEG-IFN/ ribavirin). The procedure consisted of 12-week excellent therapy administered subcutaneously with average weekly doses for 5 weeks then used for 2 months for one dose monthly of GI5005 vaccine. Previously non-responder acquired 72 weeks of excellent treatment but treatment-experienced patients acquired 48 weeks. For immunological feedback, no statistics have been issued. However, in those homozygous for the IFN-l3 risk alleles, the researcher saw a rise in persistent virological response rates [40]. In a Phase I investigation, healthy volunteers received a vaccination containing the conserved HCV core protein along with an adjuvant made of phospholipid, cholesterol, and saponin (named ISCOMATRIX®). The vaccination proved to be innocuous and among the volunteers who received the highest dose (50 µg), there was a specific humoral response to the core protein. Consequently, HCV-specific CD8+ T cells have been seen in the two patients. To analyze this strategy as a therapeutic vaccine further trial are intended by the same researcher among the HCV-infected patients. One is the feasible method to observe the genomic proteins functions of HCV for the appropriate vaccine to target this virus, the distinct properties of these proteins are shown in the Table 2.
Vector vaccines
The intriguing possibility of spreading HCV RNA via viral vectors is present. In the chimpanzee model, adenoviral vectors have been demonstrated to potently generate HCV-specific T-cell feedback and to lower the peak HCV load during the initial infection [41]. The vaccine's immunogens are not HLA constrained; hence this strategy might affect viral epitopes more than the peptide-based strategy. Modified vaccinia Ankara (MVA), a highly attenuated strain of the poxvirus, has been safely used in a number of vaccine approaches, including those for melanoma, HIV, TB, and colorectal cancer. The primary conclusions from the 2009 European Association of Liver Disease meeting [42]. Nine of the fifteen chronically infected HCV patients had a fourth treatment six months after the first three weekly injections. The HCV viral load decreased in six out of the fifteen patients (0.5–1.4 log10), and there was also a notable CD8+ T-cell feedback. Constructed a phase II trial that contrasts the immunization with conventional therapy [43]. In the Phase I vaccination study, NS HCV proteins (NS3–5B) are also being transferred to 36 healthy volunteers using adenovirus vectors. In order to further improve vaccination safety, the polymerase activity of the NS proteins is deactivated and genetically engineered vaccine vectors are used, rendering them incapable of replicating [44]. Ad6 and the simian vector AdCh3, which are used to manage the challenges of pre-existing anti-adenoviral antibodies that may limit vector efficiency, are uncommonly seen in humans. The American Association of Liver Disease published main results from this investigation in 2009, explaining that the particular priming injection that this technique ensures is highly immunogenic in healthy volunteers (Ad6) [45]. Advanced studies are planned with HCV-positive subjects.
Virus-like particles
Virus-like particles (VLPs) have been extensively researched as a potential HCV vaccine and are an attractive avenue for vaccine development [46]. As of right present, the VLP approach is being used commercially for both the HPV and the hepatitis B virus (HBV) vaccines. Virus-like particles (VLPs) are produced in a genome-independent manner when the structural proteins of a virus assemble to create a particle that mimics the virus but cannot replicate. Due to their intricate structure and ability to drain into lymph nodes, VLPs exhibit greater immunogenicity compared to soluble proteins [47]. Baumert et al. were the first to describe HCV-LPs, noting their appearance in the insect cell line Sf9 following transduction with a recombinant baculovirus containing HCV core, E1, and E2 structural proteins [48]. These HCV-LPs were found to induce the production of HCV-specific IgG, IFN-γ-secreting CD4+ T cells, and CD8+ T cell responses in both mice and baboons [49]. In a study, all four chimpanzees that received four doses of HCV-LPs exhibited CD4+ and CD8+ T cell responses. When later challenged with homologous HCV, vaccinated animals demonstrated lower viral loads compared to controls, two of which tested negative for HCV for up to two years post-challenge [50]. However, in three of these animals, humoral feedback in chimpanzees was not observed and seems to have been missing during the decrease of viremia. Since this initial trial in chimpanzees, further research on this approach has not been developed. Therefore, it is an ideal approach for upcoming HCV vaccine research, as is expressed using an experimental VLP vaccine approach the viral clearance can be accomplished. Despite work on this approach stopping, HCV-LPs persistently are to be examined. Recently, an HCV-LP method has been recognized through the recombinant adenovirus encrypting the structural genes of HCV with transduction of the human hepatoma cell line Huh 7 [51]. Primarily intended using the H77 isolate, advance progress has extended by producing HCV-LPs using the important sequences of dissimilar isolates demonstrating Gt1b, 2a and 3a subtypes to generate HCV-LPs with a configuration close to that the wild type virus [52]. Experiments of this approach in mice indicated the stimulation of nAbs and triggering of both CD4+ and CD8+ T cell feedback and recently this trial was also observed in vaccinated Landrace pigs [53]. As yet, humoral feedback analysis has illustrated that this vaccine provokes nAbs with undertaking towards homologous strains. For this approach, heterologous neutralisation data have not been described. Immunized animals with this quadrivalent vaccine against the different genetic variants of HCV after sera analysis from animals would be of special interest not only for this approach but to update upcoming vaccine programs.
DNA vaccine
A very new vaccination technology is the DNA-based immunization strategy. Compared to other common vaccinations like live attenuated viruses and recombinant protein vaccines, DNA vaccines have a far higher efficacy [54]. Numerous advantages of DNA-based vaccination include the ability to produce the vaccine, the ease with which DNA may be modified, and the immunological response that comes primarily from several sources, such as helper T cells, CTLs, and antibody feedback. Additionally, DNA vaccines are suitable for progressive immunization since early-existing antibody titers to the vector do not control their function [55]. The genetically varied HCV virus poses a significant challenge to the creation of an HCV vaccination [56]. Since many researchers have concentrated on the specific CTL feedback initiated by C and NS3 region proteins and defensive antibodies triggered by HCV E proteins, the NS3 gene is moderately conserved and plays a significant role in HCV elimination by influencing specific T cell feedback. This makes it an appropriate approach for T cell-based vaccines [57]. The HCV core should be harmed by the application of a particular vaccination under the influence of immunological response. It is a strong immunogen with anti-core immune feedback at the early stages of infection [58]. Since the HCV core protein is the most highly conserved region of the translated HCV genome throughout the many HCV genotypes, it may be the observable route for a therapeutic T-cell-based vaccination. However, different studies expressed that adaptive and innate immune responses can interfere with the core protein [59]. DNA-based vaccines substandard as compared to the conventional vaccines like subunit vaccine since the DNA vaccines concentration to regulate the immune feedback has been comparatively weak, thus efforts are focused towards the generation of novel technologies such as co-delivery of unique cytokine IL-18, IL-15, IL-12, IL-7 and IL-2 adjuvants for evading this limitation [60]. Among the current development in viral infections, HCV identification is the most significant. Researchers into the generation of new therapeutic approaches are emphasized on the study of molecular effects of the virus due to the clinical consequence of the disease. An effectual HCV vaccine should regulate the different features of the immune system such as CTL, humoral and T helper feedback. Due to the greater HCV genetic diversity and mutagenicity, developing a therapeutic or prophylactic vaccine for HCV is still a major issue. Earlier studies explained that the cellular immune responses might be crucial for an effective vaccine. Human phase I/II clinical trials are under underway for a number of HCV vaccine approaches, including vector-based, DNA, recombinant protein, and peptide vaccines, all of which have been demonstrated to give a number of benefits. It can be challenging to assess these techniques' efficacy in diseased or at-risk populations, despite the fact that many of them offer both healthy and infected volunteers’ substantial immunity.
Table 2. Hepatitis C virus genomic proteins functions. |
|
Protein |
Functions |
E1 and E2 |
Design envelope glycoprotein |
P7 |
Polyprotein maturation, viral association |
NS3 |
Helicase action |
NS2/NS3 |
Protease action |
NS4B |
Replication complex |
NS3/NS4A |
Serine protease action |
NS5B |
Development of replication complex |
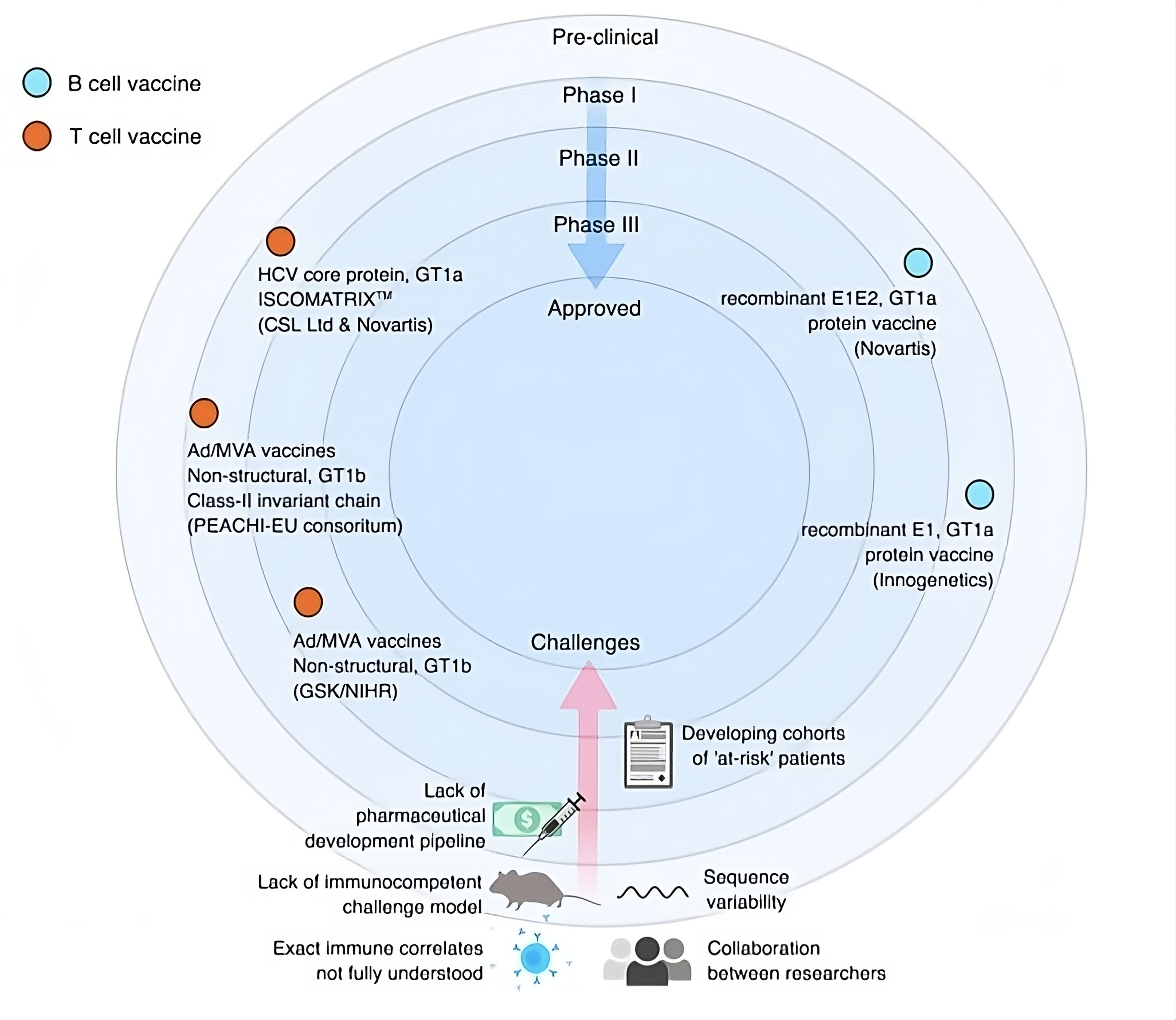
Table 3. Challenges and solutions in HCV vaccine development. |
|
Challenges in HCV vaccine development |
Clarification |
Viral genetic variability |
I. Develop mixed or multiple genotype vaccine II. Genotype specific vaccine targeting to that region where genotype dominates III. For multiple genotype design vaccine with novel immunogens |
Lack of animal models |
I. Development in the novel small animal models II. Speculation in phase- I/ II human research |
Lack of human challenge models |
Could be considered now |
For phase-II/III studies well characterized cohorts |
Financial assistance for cohort studies |
Lack of pharmaceutical investment |
To ensure funding by government and non-government organizations |
No applicable.
Ethics approval
No applicable.
Data availability
The data will be available upon request.
Funding
None.
Authors’ contribution
Muhammad Shoaib Akhtar contributed to draft and critical revision of the article and approved the final version to be submited.
Competing interests
None.
- Organization WH: Global hepatitis report 2017: World Health Organization; 2017.
- de Franchis R: Revising consensus in portal hypertension: report of the Baveno V consensus workshop on methodology of diagnosis and therapy in portal hypertension. J Hepatol 2010, 53(4): 762-768.
- Falade-Nwulia O, Suarez-Cuervo C, Nelson DR, Fried MW, Segal JB, Sulkowski MS: Oral direct-acting agent therapy for hepatitis C virus infection: a systematic review. Ann Intern Med 2017, 166(9): 637-648.
- Rice CM, Saeed M: Treatment triumphs. Nature 2014, 510(7503): 43-44.
- Gravitz L: Introduction: Next generation biofuels. Nature 2011, 474(7352): S2-S5.
- Cox AL: Global control of hepatitis C virus. Science 2015, 349(6250): 790-791.
- Thomas DL: Global control of hepatitis C: where challenge meets opportunity. Nature Med 2013, 19(7): 850-858.
- Wu H-H, Chen Y-T, Shih C-J, Lee Y-T, Kuo S-C, Chen T-L: Association between recent use of proton pump inhibitors and nontyphoid salmonellosis: a nested case-control study. Clin Infect Dis 2014, 59(11): 1554-1558.
- Thursz M, Fontanet A: HCV transmission in industrialized countries and resource-constrained areas. Nat Rev Gastroenterol Hepatol 2014, 11(1): 28-35.
- Falade‐Nwulia O, Sulkowski MS, Merkow A, Latkin C, Mehta SH: Understanding and addressing hepatitis C reinfection in the oral direct‐acting antiviral era. J Viral Hepat 2018, 25(3): 220-227.
- Habib A, Razzak KSB, Imran M, Khalid B: Elimination of Hepatitis C Virus: A Goal of WHO. Vigyan Varta 2020, 1: 56-59.
- Gvinjilia L, Nasrullah M, Sergeenko D, Tsertsvadze T, Kamkamidze G, Butsashvili M, Gamkrelidze A, Imnadze P, Kvaratskhelia V, Chkhartishvili N et al: National progress toward hepatitis C elimination-Georgia, 2015-2016. Morbidity and Mortality Weekly Report. 2016, 65(41): 1132-1135.
- Plotkin S, Fine P, Eames K, Heyman D: "Herd immunity": a rough guide. Clin Infect Dis 2001, 52(7): 911-916.
- Frey SE, Houghton M, Coates S, Abriganani S, Chien D, Rosa D, Pileri P, Ray R, Bisceglie AMD, Rinella P et al: Safety and immunogenicity of HCV E1E2 vaccine adjuvanted with MF59 administered to healthy adults. Vaccine 2010, 28(38): 6367-6373.
- Blach S, Zeuzem S, Manns M, Altraif I, Duberg AS, Muljono DH, Waked I, Alavian SM, Lee MH, Negro F et al: Global prevalence and genotype distribution of hepatitis C virus infection in 2015: a modelling study. Lancet Gastroenterol Hepatol 2017, 2(3): 161-176.
- Sepulveda-Crespo D, Resino S, Martinez I: Hepatitis C virus vaccine design: focus on the humoral immune response. J Biom Sci 2020, 27(1): 1-12.
- Westbrook R, Dusheiko G: Natural history of hepatitis CJ Hepatol 61 (Suppl. 1). S58-S68 2014.
- Sepulveda-Crespo D, Resino S, Martinez I: Innate immune response against hepatitis C virus: targets for vaccine adjuvants. Vaccines 2020, 8(2): 313.
- Lingala S, Ghany MG: Natural history of hepatitis C. Gastroenterol Clin 2015, 44(4): 717-734.
- Burton DR, Poignard P, Stanfield RL, Wilson IA: Broadly neutralizing antibodies present new prospects to counter highly antigenically diverse viruses. Science 2012, 337(6091): 183-186.
- Haynes BF, Gilbert PB, McElrath MJ, Zolla-Pazner S, Tomaras GD, Alam SM, Evans DT, Montefiori DC, Karnasuta C, Sutthent R et al: Immune-correlates analysis of an HIV-1 vaccine efficacy trial. N Engl J Med 2012, 366(14): 1275-1286.
- Raghuraman S, Park H, Osburn WO, Winkelstein E, Edlin BR, Rehermann B: Spontaneous clearance of chronic hepatitis C virus infection is associated with appearance of neutralizing antibodies and reversal of T-cell exhaustion. J Infect Dis 2012, 205(5): 763-771.
- Pestka JM, Zeisel MB, Bläser E, Schürmann P, Bartosch B, Cosset FL, Patel AH, Meisel H, Baumert J, Viazov S et al: Rapid induction of virus-neutralizing antibodies and viral clearance in a single-source outbreak of hepatitis C. Proc Nat Acad Sci 2007, 104(14): 6025-6030.
- Baumert TF, Wellnitz S, Aono S, Satoi J, Herion D, Gerlach TJ, Pape GR, Lau JY, Hoofnagle JH, Blum HE et al: Antibodies Against Hepatitis C Virus–Like Particles and Viral Clearance in Acute and Chronic Hepatitis C. Hepatol 2000, 32(3): 610-617.
- Houghton M: Prospects for prophylactic and therapeutic vaccines against the hepatitis C viruses. Immunol Rev 2011, 239(1): 99-108.
- Farci P, Alter HJ, Govindarajan S, Wong DC, Engle R, Lesniewski RR, Mushahwar IK, Desai SM, Miller RH, Ogata N et al: Lack of protective immunity against reinfection with hepatitis C virus. Science 1992, 258(5079): 135-140.
- Meunier J-C, Russell RS, Goossens V, Priem S, Walter H, Depla E, Union A, Faulk KN, Bukh J, Emerson SU et al: Isolation and characterization of broadly neutralizing human monoclonal antibodies to the e1 glycoprotein of hepatitis C virus. J Virol 2008, 82(2): 966-973.
- Verstrepen BE, Depla E, Rollier CS, Mares G, Drexhage JA, Priem S, Verschoor EJ, Koopman G, Granier C, Dreux M et al: Clearance of genotype 1b hepatitis C virus in chimpanzees in the presence of vaccine-induced E1-neutralizing antibodies. J Infect Dis 2011, 204(6): 837-844.
- Meunier J-C, Gottwein JM, Houghton M, Russell RS, Emerson SU, Bukh J, Purcell RH et al: Vaccine-induced cross-genotype reactive neutralizing antibodies against hepatitis C virus. J Infect Dis 2011, 204(8): 1186-1190.
- Law JLM, Chen C, Wong J, Hockman D, Santer DM, Frey SE, Belshe RB, Wakita T, Bukh J, Jones CT et al: A hepatitis C virus (HCV) vaccine comprising envelope glycoproteins gpE1/gpE2 derived from a single isolate elicits broad cross-genotype neutralizing antibodies in humans. PloS one 2013, 8(3): e59776.
- Brimacombe CL, Grove J, Meredith LW, Hu K, Syder AJ, Flores MV, Timpe JM, Krieger SE, Baumert TF, Tellinghuisen TL, et al: Neutralizing antibody-resistant hepatitis C virus cell-to-cell transmission. J Virol 2011, 85(1): 596-605.
- Fofana I, Xiao F, Thumann C, Turek M, Zona L, Tawar RG, Grunert F, Thompson J, Zeisel MB, Baumert TF et al: A novel monoclonal anti-CD81 antibody produced by genetic immunization efficiently inhibits Hepatitis C virus cell-cell transmission. PloS one 2013, 8(5): e64221.
- Walker CM: Adaptive immunity to the hepatitis C virus. Adv Virus Res 2010, 78: 43-86.
- Drane D, Maraskovsky E, Gibson R, Mitchell S, Barnden M, Moskwa A, Shaw D, Gervase B, Coates S, Houghton M et al: Priming of CD4+ and CD8+ T cell responses using a HCV core ISCOMATRIX™ vaccine: A phase I study in healthy volunteers. Human Vaccines 2009, 5(3): 151-157.
- Habersetzer F, Honnet G, Bain C, Maynard-Muet M, Leroy V, Zarski JP, Feray C, Baumert TF, Bronowicki JP, Doffoël M et al: A poxvirus vaccine is safe, induces T-cell responses, and decreases viral load in patients with chronic hepatitis C. Gastroenterol 2011, 141(3): 890-899. e894.
- Wedemeyer H, Janczewska E, Wlodzimierz M, Stanciu C, Habersetzer F, Carreno V, Di Bisceglie AM, Tanasescu C, Flisiak R, Romero-Gomez M et al: 1403 Significant improvement of complete EVR in HCVAC phase II clinical trial when adding TG4040 therapeutic vaccine to peg ifnα2A and ribavirin. J Hepatol 2012, 56: S552.
- Man John Law L, Landi A, Magee WC, Lorne Tyrrell D, Houghton M: Progress towards a hepatitis C virus vaccine. Emerg Microb Infect 2013, 2(1): 1-7.
- Levander S: Development of vaccines and mouse models for chronic hepatitis C virus infection: Inst för laboratoriemedicin/Dept of Laboratory Medicine; 2016.
- Habersetzer F, Baumertºº TF, Stoll-Keller F: Gl-5OO5, a yeast vector vaccine expressing an NS3-core fusion protein. Curr Opin Mol Ther 2009, 11(4): 456-462.
- McHutchison J, Goodman Z, Everson G, Jacobson IM, Boyer TD, Schiff ER, Lee WM, Chasen RM, Vierling JM, Lawitz10 EJ et al: GI-5005 therapeutic vaccine plus peg-IFN/ribavirin improves biopsy necro-inflammatory scores and ALT normalization at 48 weeks versus peg-IFN/ribavirin in genotype 1 chronic HCV patients. J Hepatol 2010, 52: S116.
- Folgori A, Capone S, Ruggeri L, Meola A, Sporeno E, Ercole BB, Pezzanera M, Tafi R, Arcuri M, Fattori E et al: A T-cell HCV vaccine eliciting effective immunity against heterologous virus challenge in chimpanzees. Nature Med 2006, 12(2): 190-197.
- Habersetzer F, Zarski J-P, Leroy V, Maynard-Muet M, Bronowicki JP, Feray C, Hézode C, Fournillier A, Bain C, Inchauspé G et al: 42 A novel vectorized HCV therapeutic vaccine (TG4040): results of a phase I study in naïve patients chronically infected by HCV. J Hepatol 2009, 50: S18.
- Halliday J, Klenerman P, Barnes E: Vaccination for hepatitis C virus: closing in on an evasive target. Exper Rev Vaccines 2011, 10(5): 659-672.
- Roohvand F, Kossari N: Advances in hepatitis C virus vaccines, part two: advances in hepatitis C virus vaccine formulations and modalities. Exper Opin Ther 2012, 22(4): 391-415.
- Ghasemi F, Rostami S, Meshkat Z: Progress in the development of vaccines for hepatitis C virus infection. World J Gastroenterol 2015, 21(42): 11984.
- Torresi J: The rationale for a preventative HCV virus-like particle (VLP) vaccine. Front Microbiol 2017, 8: 2163.
- Bachmann MF, Jennings GT: Vaccine delivery: a matter of size, geometry, kinetics and molecular patterns. Nat Rev Immunol 2010, 10(11): 787-796.
- Baumert TF, Ito S, Wong DT, Liang TJ: Hepatitis C virus structural proteins assemble into viruslike particles in insect cells. J Virol 1998, 72(5): 3827-3836.
- Jeong S-H, Qiao M, Nascimbeni M, Hu Z, Rehermann B, Murthy K, Liang TJ: Immunization with hepatitis C virus-like particles induces humoral and cellular immune responses in nonhuman primates. J Virol 2004, 78(13): 6995-7003.
- Elmowalid GA, Qiao M, Jeong SH, Borg BB, Baumert TF, Sapp RK, Hu Z, Murthy K, Liang TJ: Immunization with hepatitis C virus-like particles results in control of hepatitis C virus infection in chimpanzees. Proc Nat Acad Sci 2007, 104(20): 8427-8432.
- Chua BY, Johnson D, Tan A, Earnest-Silveira L, Sekiya T, Chin R, Torresi J, Jackson DC: Hepatitis C VLPs delivered to dendritic cells by a TLR2 targeting lipopeptide results in enhanced antibody and cell-mediated responses. PloS one 2012, 7(10): e47492.
- Collett S, Torresi J, Earnest-Silveira L, Christiansen D, Elbourne A, Ramsland PA: Probing and pressing surfaces of hepatitis C virus-like particles. J Colloid Interface Sci 2019, 545: 259-268.
- Christiansen D, Earnest-Silveira L, Grubor-Bauk B, Wijesundara DK, Boo I, Ramsland PA, Vincan E, Drummer HE, Gowans EJ, Torresi J et al: Pre-clinical evaluation of a quadrivalent HCV VLP vaccine in pigs following microneedle delivery. Sci Rep 2019, 9(1): 1-13.
- Lang KA, Yan J, Draghia-Akli R, Khan A, Weiner DB: Strong HCV NS3-and NS4A-specific cellular immune responses induced in mice and Rhesus macaques by a novel HCV genotype 1a/1b consensus DNA vaccine. Vaccine 2008, 26(49): 6225-6231.
- Randal J: Hepatitis C vaccine hampered by viral complexity, many technical restraints. J Natl Cancer Inst 1999, 91(11): 906-908.
- Naderi M, Saeedi A, Moradi A, Kleshadi M, Zolfaghari MR, Gorji A, Ghaemi A: Interleukin-12 as a genetic adjuvant enhances hepatitis C virus NS3 DNA vaccine immunogenicity. Virol Sin 2013, 28(3): 167-173.
- Tang LL, Liu KZ: Recent advances in DNA vaccine of hepatitis virus. Hepatobiliary Pancreat Dis Int 2002, 1(2): 228-231.
- Alekseeva E, Sominskaya I, Skrastina D, Egorova I, Starodubova E, Kushners E, Mihailova M, Petrakova N, Bruvere R, Kozlovskaya T et al: Enhancement of the expression of HCV core gene does not enhance core-specific immune response in DNA immunization: advantages of the heterologous DNA prime, protein boost immunization regimen. Genet Vaccines Ther 2009, 7(1): 1-17.
- Sarobe P, Lasarte JJ, Zabaleta A, Arribillaga L, Arina A, Melero I, Borrás-Cuesta F, Prieto J: Hepatitis C virus structural proteins impair dendritic cell maturation and inhibit in vivo induction of cellular immune responses. J Virol 2003, 77(20): 10862-10871.
- Choi SY, Suh YS, Cho JH, Jin HT, Chang J, Sung YC: Enhancement of DNA vaccine-induced immune responses by influenza virus NP gene. Immune Net 2009, 9(5): 169-178.
- Li Y, Pierce BG, Wang Q, Keck ZY, Fuerst TR, Foung SK, Mariuzza RA: Structural basis for penetration of the glycan shield of hepatitis C virus E2 glycoprotein by a broadly neutralizing human antibody. J Biol Chem 2015, 290(16): 10117-10125.
- Keck ZY, Angus AG, Wang W, Lau P, Wang Y, Gatherer D, Patel AH, Foung SK: Non-random escape pathways from a broadly neutralizing human monoclonal antibody map to a highly conserved region on the hepatitis C virus E2 glycoprotein encompassing amino acids 412-423. PLoS Pathog 2014, 10(8): e1004297.
- Pierce BG, Keck ZY, Wang R, Lau P, Garagusi K, Elkholy K, Toth EA, Urbanowicz RA, Guest JD, Agnihotri P, Kerzic MC: Structure-based design of hepatitis C virus E2 glycoprotein improves serum binding and cross-neutralization. J Virol 2020, 94(22): 10-128.
- Wijesundara DK, Gummow J, Li Y, Yu W, Quah BJ, Ranasinghe C, Torresi J, Gowans EJ, Grubor-Bauk B: Induction of genotype cross-reactive, hepatitis C virus-specific, cell-mediated immunity in DNA-vaccinated mice. J Virol 2018, 92(8): e02133-17.
- Marín MQ, Pérez P, Ljungberg K, Sorzano CÓ, Gómez CE, Liljeström P, Esteban M, García-Arriaza J: Potent anti-hepatitis C virus (HCV) T cell immune responses induced in mice vaccinated with DNA-launched RNA replicons and modified vaccinia virus Ankara-HCV. J Virol 2019, 93(7): e00055-19.
- Masavuli MG, Wijesundara DK, Underwood A, Christiansen D, Earnest-Silveira L, Bull R, Torresi J, Gowans EJ, Grubor-Bauk B: A hepatitis C virus DNA vaccine encoding a secreted, oligomerized form of envelope proteins is highly immunogenic and elicits neutralizing antibodies in vaccinated mice. Front Immunol 2019, 10: 1145.
- Dawood RM, Moustafa RI, Abdelhafez TH, El-Shenawy R, El-Abd Y, Bader El Din NG, Dubuisson J, El Awady MK: A multiepitope peptide vaccine against HCV stimulates neutralizing humoral and persistent cellular responses in mice. BMC Infect Dis 2019, 19(1): 1-11.
- Filskov J, Andersen P, Agger EM, Bukh J: HCV p7 as a novel vaccine-target inducing multifunctional CD4+ and CD8+ T-cells targeting liver cells expressing the viral antigen. Sci Rep 2019, 9(1): 1-13.
- Swadling L, Capone S, Antrobus RD, Brown A, Richardson R, Newell EW, Halliday J, Kelly C, Bowen D, Fergusson J et al: A human vaccine strategy based on chimpanzee adenoviral and MVA vectors that primes, boosts, and sustains functional HCV-specific T cell memory. Sci Transl Med 2014, 6(261): 261ra153-261ra153.
- Wei S, Lei Y, Yang J, Wang X, Shu F, Wei X, Lin F, Li B, Cui Y, Zhang H et al: Neutralization effects of antibody elicited by chimeric HBV S antigen viral-like particles presenting HCV neutralization epitopes. Vaccine 2018, 36(17): 2273-2281.
- Beaumont E, Patient R, Hourioux C, Dimier‐Poisson I, Roingeard P: Chimeric hepatitis B virus/hepatitis C virus envelope proteins elicit broadly neutralizing antibodies and constitute a potential bivalent prophylactic vaccine. Hepatology 2013, 57(4): 1303-1313.
- Vietheer PT, Boo I, Gu J, McCaffrey K, Edwards S, Owczarek C, Hardy MP, Fabri L, Center RJ, Poumbourios P et al: The core domain of hepatitis C virus glycoprotein E2 generates potent cross‐neutralizing antibodies in guinea pigs. Hepatology 2017, 65(4): 1117-1131.
- Duncan JD, Urbanowicz RA, Tarr AW, Ball JK: Hepatitis C virus vaccine: challenges and prospects. Vaccines 2020, 8(1): 90.
- Donnison T, Chinnakannan S, Cicconi P, Barnes E: Hepatitis C vaccines: how close are we to the promised land ? Clinical Dilemmas in Viral Liver Disease 2020, 208-215.
Asia-Pacific Journal of Pharmacotherapy & Toxicology
p-ISSN: 2788-6840
e-ISSN: 2788-6859
Copyright © Asia Pac J Pharmacother Toxicol. This work is licensed under a Creative Commons Attribution-NonCommercial-No Derivatives 4.0 International (CC BY-NC-ND 4.0) License.