Research Article | Open Access
Antigenic switch potential of influenza D virus
Abdul Bari Hejran1, 2, *, Khaidarov Saken2, *, Rahmatullah Afghan3, Parwiz Niazi4
1Department of Biology, Faculty of Education, Helmand University, Helmand, Afghanistan.
2Department of Biotechnology, Faculty of Biology and Biotechnology, Al-Farabi Kazakh National University, Almaty, Kazakhstan.
3Department of Physics, Faculty of Education, Helmand University, Helmand, Afghanistan.
4Department of Biology, Faculty of Education, Kandahar University, Kandahar, Afghanistan.
Correspondence: Abdul Bari Hejran (Department of Biology, Faculty of Education, Helmand University, Lashkar Gah, 3901, Afghanistan; Department of Biotechnology, Faculty of Biology and Biotechnology, Al-Farabi Kazakh National University, Almaty 050040, Kazakhstan; E-mail: abdulbari.hejran94@gmail.com) and Khaidarov Saken (Department of Biotechnology, Faculty of Biology and Biotechnology, Al-Farabi Kazakh National University, Almaty 050040, Kazakhstan; Email: logicalmind1984@mail.ru).
Asia-Pacific Journal of Pharmacotherapy & Toxicology 2024, 4: 85-94. https://doi.org/10.32948/ajpt.2024.11.25
Received: 30 Oct 2024 | Accepted: 25 Nov 2024 | Published online: 01 Dec 2024
Key words influenza D virus, antigenic switch, hemagglutinin-esterase fusion, zoonotic potential, host immunogenicity
Furthermore, the binding characteristics of Influenza D exhibit variability across different virus clades and wildlife species. A tissue microarray system was employed to investigate the respiratory tissues of wild and domestic animals, demonstrating that IDV can adhere to the respiratory tracts of wildlife, thereby potentially promoting transmission [23, 24]. The influenza D virus is closely related to the human influenza C virus. It is suggested to be a natural reservoir in cattle, potentially contributing to the bovine respiratory disease complex. While the pathogenic potential of IDV in humans remains largely unexplored, it poses a significant risk as an emerging pathogen for individuals working with cattle. IDV exhibits a broad host range and can cross species barriers. Emerging threats from influenza A viruses (IAVs) arise from genetic drift and shift, resulting in frequent epidemics and the necessity for annual vaccine reformulations. Pigs, quails, and bats serve as mixing vessels for IAVs, as they co-express both SAα2,3-Gal and SAα2,6-Gal receptors, which are essential for binding to avian and human IAVs [25, 26, 27, 28, 29, 30, 31].
Finally, the influenza D virus has been increasingly detected in cattle and swine populations across the globe, demonstrating exceptional thermal and acid stability along with a broad host range. Cattle have been identified as the natural reservoir and amplification host for IDV, with infections contributing to mild or moderate respiratory illnesses in cattle and playing a significant role in the bovine respiratory disease (BRD) complex. This complex is the most common and economically impactful cattle industry disease. Recent advances in understanding the biology and epidemiology of IDV have highlighted its pathogenesis and potential zoonotic risks. Antibodies specific to IDV have been found in several species beyond cattle and swine, including horses, small ruminants, feral swine, buffalo, camels, and humans, particularly among those exposed to cattle. These findings underline the increasing significance of IDV as a pathogen with potential interspecies transmission. These considerations will further elaborate on various critical aspects of influenza viruses, such as the antigenic shift across all types, the agricultural theory behind IDV, and vaccination and epidemiological studies. The role of swine as intermediate vectors, along with treatment, prevention strategies, vaccine development, and prospects, will also be discussed, offering a broad and diverse range of insights into these pressing issues Table 1 [32, 33, 26, 3, 4, 24, 34, 35, 36, 37, 38, 6, 39, 40].
Table 1. Exploring the complex effects and mitigation approaches of influenza d virus across diverse biological systems. |
||||||||
Target tissues |
Description |
Control strategies |
Vaccine (year, country) |
Negative/Positive parameters |
Clinical phase |
Mechanism of action |
Future prospects |
References |
Respiratory Tract (e.g., lungs, trachea) |
Influenza D virus primarily targets the respiratory epithelium, causing respiratory illnesses in cattle, swine, and other animals. Viral replication leads to inflammation and damage to respiratory tissues. |
Biosecurity: Improved biosecurity in farms. Vaccination: Development of species-specific vaccines. Surveillance: Enhanced global livestock and intermediate vectors surveillance to monitor viral mutations. |
Ongoing research (no licensed vaccines yet; vaccine trials in development globally). |
Positive: Shows antigenic variation, expanding host range. Negative: Cross-species transmission poses risks for zoonotic spillover—potential for reassortment with other viruses, increasing its adaptation to new hosts. |
Preclinical (animal models) |
Hemagglutinin-esterase fusion protein binds to sialic acid receptors, facilitating viral entry and replication in respiratory epithelial cells. Mutations enhance receptor-binding efficiency and immune evasion. |
Cross-species transmission: Future research may focus on the development of multi-species vaccines. Surveillance: Global monitoring for mutations and viral evolution that could enhance zoonotic potential and the virus's adaptability to new environments. |
[41, 42, 43]. |
Gastrointestinal Tract |
Some studies suggest that IDV may target the gastrointestinal tract, particularly in cattle, causing mild symptoms. Gastrointestinal involvement is still under investigation. |
Hygiene and Sanitation: Reducing fecal-oral transmission through improved sanitation in farming environments. Antivirals: Testing potential antiviral drugs targeting viral replication. |
It is in progress (various trials in cattle and swine). |
Positive: Reduced pathogenicity in gastrointestinal infections. Negative: Limited understanding of the virus's replication in non-respiratory tissues may lead to underestimating transmission routes. |
Experimental |
Viral replication in gastrointestinal tissues may rely on similar mechanisms to respiratory replication, particularly receptor-mediated entry into epithelial cells. |
Antiviral Treatments: Future antiviral therapies could target respiratory and gastrointestinal infections to mitigate disease spread. Mutagenesis Studies: Understanding how mutations influence tissue tropism could open new therapeutic avenues. |
[6, 44]. |
Immune System (Innate and Adaptive) |
Mutations in the hemagglutinin-esterase fusion protein allow the virus to evade immune responses and avoid detection by neutralizing antibodies. |
Immune Modulation: Use of immunomodulatory therapies to boost innate immunity in affected animals. Adjuvant Vaccines: Development of vaccines that enhance adaptive immune responses to overcome immune evasion mechanisms. |
Development stage in North America (2022 trials in cattle). |
Positive: Moderate stimulation of innate immune responses in early infection. Harmful: Evasion of adaptive immune responses through antigenic variation, leading to long-term immunity and vaccine efficacy challenges. |
Phase I (vaccine trials) |
Mutations in vital antigenic sites allow the virus to escape neutralizing antibodies, while altered surface proteins enhance immune evasion. This allows persistent infection or rapid spread in immunologically naïve populations. |
Vaccine Enhancement: Future vaccines may incorporate novel adjuvants to improve immunogenicity. Genetic Engineering: Potential for using reverse genetics to produce attenuated strains that stimulate more robust immune responses. |
[45, 46]. |
Endothelial Cells |
IDV may infect endothelial cells, contributing to vascular inflammation and exacerbating respiratory symptoms. Although not fully established, endothelial involvement is suspected in severe cases. |
Anti-inflammatory Strategies: Investigating anti-inflammatory drugs to minimize vascular damage. Prophylactic Antiviral Use: Use broad-spectrum antivirals in herds experiencing outbreaks to limit viral spread. |
There is no current vaccine. Early research into endothelial effects is ongoing. |
Positive: Vascular involvement may increase understanding of severe cases. Damaging: The lack of confirmed data on endothelial cell infection limits the ability to develop targeted therapies. |
Exploratory studies |
Potential infection of endothelial cells could contribute to vascular inflammation, though the exact mechanism of interaction between IDV and endothelial cells remains under investigation. |
Host Range Expansion: Future studies could examine the potential for IDV to affect the vascular systems of non-traditional hosts, leading to a greater understanding of severe pathogenicity in humans. |
[47, 48]. |
Neurological System (possible) |
Though rare, neurological symptoms in affected animals, such as swine, have been reported in severe cases, likely secondary to systemic infection rather than primary neural invasion. |
Neurological Surveillance: Increased monitoring of neurological symptoms during outbreaks. Anti-inflammatory Drugs: Testing anti-neuroinflammatory drugs in severe cases where neurological involvement is suspected. |
No vaccine addressing neurological impacts. |
Positive: Limited neurological involvement in most cases. Negative: Neurological symptoms may complicate treatment and prognosis in rare instances of severe systemic infection. |
Not applicable |
Secondary neurological effects may arise from systemic inflammatory responses rather than direct infection of neural tissues. The mechanisms remain unclear and require further investigation. |
Neurological Research: Studies into potential neurotropic characteristics could be vital for assessing IDV's pathogenicity in non-traditional hosts, including humans. |
[25, 49]. |
Reproductive System (in swine) |
Possible reproductive impacts on swine are under investigation. Although evidence is limited, preliminary studies suggest that IDV may have mild effects on reproductive tissues. |
Reproductive Health Monitoring: Regular monitoring of reproductive health in swine herds during IDV outbreaks. Preventive Measures: Research into vaccine development aimed at reducing reproductive effects. |
Research is in progress (no specific vaccines address reproductive impacts). |
Positive: No significant reproductive health effects have been observed so far. Negative: Limited research may underestimate the virus’s potential impact on reproduction, leading to prevention and control measures gaps. |
Preclinical (animal studies) |
Direct reproductive system infection is unlikely; reproductive impacts may be secondary to systemic disease, similar to other influenza viruses. |
Long-Term Studies: Future longitudinal studies could assess whether IDV affects reproductive health over multiple generations in livestock, particularly swine, and its possible effects on fertility and neonatal health. |
[50, 48]. |
Ocular Tissue (rare cases) |
IDV's effect on ocular tissues remains understudied, but there have been occasional reports of conjunctivitis and other mild ocular symptoms in infected animals, typically associated with respiratory distress. |
Ocular Treatment: Use of anti-inflammatory eye drops in symptomatic animals. Ocular Surveillance: Monitoring ocular symptoms in animals during outbreaks to assess correlation with respiratory infections. |
There are no current vaccines for ocular impacts (rare symptomology). |
Positive: Mild and self-limiting ocular involvement. Damaging: Lack of specific treatment protocols for ocular symptoms can lead to animal discomfort during respiratory infections. |
There is no particular phase for ocular involvement |
Mild ocular symptoms may arise from inflammatory responses associated with respiratory infection rather than direct ocular infection by the virus. |
Ocular Involvement: Further studies could clarify whether IDV can directly infect ocular tissues or whether observed symptoms are purely secondary to systemic respiratory inflammation. |
[51, 52]. |
Cardiovascular System |
Cardiovascular effects are suspected but not well-documented in IDV infections. In cases of severe respiratory distress, secondary cardiovascular symptoms may arise due to systemic inflammation. |
Cardiovascular Monitoring: Enhanced monitoring of cardiovascular health during outbreaks. Anti-inflammatory Drugs: Testing of cardiovascular-targeted treatments in severe systemic infections to reduce inflammation. |
No current vaccine addressing cardiovascular impacts (limited research). |
Positive: Rare cardiovascular symptoms observed. Negative: In cases of severe systemic infection, cardiovascular involvement could exacerbate morbidity and complicate treatment efforts. |
No clinical trials for cardiovascular effects yet |
Systemic inflammation associated with severe respiratory infections could lead to cardiovascular symptoms, although direct infection of cardiovascular tissues is unlikely based on current evidence. |
Future Research: Studies into the potential secondary cardiovascular effects could improve understanding of severe cases, particularly in livestock with pre-existing cardiovascular conditions, and inform treatment strategies. |
[41, 54, 55]. |
Antigenic shift of all types of influenza viruses
The neuraminidase enzyme associated with influenza.Neuraminidase, a type II membrane-anchored glycoprotein on the surface of influenza virions, is essential in ensuring adequate protection. Nonetheless, neuraminidase's limited potency and stability in existing influenza vaccines present significant obstacles to eliciting robust immune responses. Strategies such as isolating and characterizing broadly reactive monoclonal antibodies against neuraminidase have been explored to augment anti-neuraminidase immune reactions. The catalytic site located within the head domain of each promoter is highly conserved and serves as a target for antiviral drug and monoclonal antibody development. Cathode ions are critical for enzymatic activity and thermal stability, while the architecture of the neuraminidase stalk domain exhibits variability. Variations in the neuraminidase globular head exposure can significantly influence viral infectivity, transmission, and immunogenicity. Furthermore, the surface of the neuraminidase protein may be extensively glycosylated, contributing to its structural integrity, functionality, specificity, thermal stability, antigenic properties, and immunogenic potential. Removing glycans from the neuraminidase apex enhances protective efficacy [65, 66, 67, 68, 69, 70, 71, 24, 72, 73].
Subsequently, the reassortment between Swine H3N2 and the 2009 Pandemic H1N1 in the United States has resulted in influenza A viruses exhibiting a variety of genetic constellations. A study conducted from 2009 to 2016 identified 44 distinct genotypes of H3N2 influenza A viruses (IAV) in swine, with the predominant genotype (32.33%) characterized by a clade IV-A hemagglutinin (HA) gene, 2002-lineage neuraminidase (NA) gene, an M-pdm09 gene, and other gene segments derived from a triple reassortant internal gene (TRIG) source. This genetic diversity poses challenges for disease management within the swine industry. It presents potential public health risks, mainly if swine-adapted viruses containing H1N1pdm09 genes exhibit an elevated propensity for human infection [74, 75, 68, 76, 77, 78, 79, 80, 48, 81, 18, 82, 83, 84, 50]. A study has identified four H3N2 canine influenza viruses (CIVs) as a potential public health concern due to their close genetic affinity with human strains. The virus was isolated from nasal swabs of dogs in China between 2018 and 2020, and phylogenetic analysis uncovered 15 distinct genotypes, with genotype 15 dominating among dogs since 2017. Molecular characterization revealed several adaptive substitutions in mammals, including HA-G146S, HA-N188D, PB2-I292T, PB2-G590S, PB2-S714I, PB1-D154G, and NP-R293K. A novel adaptive mutation, HA-V223I, was detected in H3N2 viruses from humans and swine, indicating its potential significance in mammalian adaptation. Human H3N2 vaccines do not protect H3N2 CIVs, underscoring the necessity for vigilant surveillance and monitoring [85, 86, 76, 78, 71, 87, 88, 89, 81, 90]. Shandong Province, China, has identified 21 reassortant G4 genotype Eurasian avian-like (EA) H1N1 subtypes of swine influenza viruses (SIVs) that pose potential health risks. These viruses harbor genes from multiple lineages and possess mutation sites that may enhance mammalian fitness and pathogenicity in murine models. The study advocates for improved surveillance to evaluate the pandemic potential of these viruses. SIVs are highly contagious respiratory pathogens in pigs and can infect various animal hosts, including humans, birds, dogs, cats, horses, ferrets, minks, and seals [91, 86, 92, 79, 87, 48, 89, 84].
Antigenic Diversity Among IDVs Utilizing Immune Antisera.To investigate the antigenic variation of IDV HEF proteins, hemagglutination inhibition (HI) and viral neutralization (VN) assays were performed. These assays utilized mouse polyclonal antibodies generated against specific viral strains, including D/OK, D/660, D/Yama2016, and D/Yama2019 lineages. A recombinant virus (rCA/OK) was also tested. Results confirmed that while all antisera reacted to homologous and heterologous viral strains, reactivity to heterologous viruses exhibited reduced titers. This variation underscores potential antigenic diversity among IDV HEF lineages. Notably, homologous strains showed the highest titers, while heterologous reactions were often eight-fold lower in the HI assay and up to 16-fold lower in VN tests. This pattern highlights possible antigenic differentiation across lineages (Figure 2).
Antigenic Variation Among IDV HEFs Employing Monoclonal Antibodies. Mouse hybridomas secreting monoclonal antibodies (mAbs) against HEF antigens were produced to analyze antigenicity further. Specific mAbs were found to react either with homologous viruses or across multiple strains. These results suggested the presence of strain-specific epitopes and cross-reactive regions among IDV HEFs.
Identification of Antigenic Epitopes via Monoclonal Antibodies:.Virus neutralization escape mutants were generated to map antigenic epitopes on the HEF protein. Various amino acid substitutions were identified in escape mutants, highlighting the potential regions responsible for VN activity loss. By comparing these mutants' sequences with their wild-type counterparts, specific epitopes crucial for immune recognition were pinpointed.
Mapping of Epitopes on the Hemagglutinin-Esterase Fusion Molecule. The identified amino acid changes were plotted onto the HEF structure, revealing that critical antigenic epitopes were located near the receptor-binding region or within the esterase domain.
Commonality of Hemagglutinin-Esterase Epitopes across Viral Lineages. Some mAbs recognized epitopes in the receptor-binding region that were conserved across multiple viral lineages. This observation suggests that specific epitopes, such as positions 187 and 189, are preserved and critical for cross-lineage antigenic responses Table 2 [93, 94, 2, 14, 16, 95, 96, 97, 73, 40, 98].
Antigenic Drift and Shift in Influenza. The influenza virus undergoes antigenic evolution through two primary mechanisms: antigenic drift and antigenic shift. These processes involve changes in the virus's surface glycoproteins, specifically hemagglutinin (HA) and neuraminidase (NA), leading to the continuous need for updates in vaccine antigens to match circulating strains.
Antigenic Drift is a gradual process in which small mutations in the HA and NA proteins occur over time, resulting in minor changes to the virus. These mutations can enable the virus to evade the immune responses generated by previous infections or vaccinations, making the immune response generated by existing vaccines less effective.
Antigenic Shift. In contrast, antigenic shift involves a more significant change, typically through reassortment between different viral strains. This can lead to new virus subtypes that the human population has little to no pre-existing immunity against, potentially resulting in pandemics. The constant antigenic changes presented by both drift and shift challenge vaccine development, necessitating a system of global virus tracking and annual vaccine reformulations. The World Health Organization (WHO) is critical in monitoring these changes and selecting appropriate virus strains for inclusion in seasonal influenza vaccines [21, 99, 53, 100, 80, 101].
Epidemiology and vaccinations of influenza D virus
Influenza D virus (IDV) is a significant cause of the bovine respiratory disease complex (BRDC), the cattle industry's most common and costly ailment. A recombinant strain (rD/OK-AL) was engineered to create a candidate vaccine using mutations in the PB2 and PB1 proteins to enhance cold adaptation and high-temperature sensitivity. This strain grew effectively at 33°C but not at 37°C. In mouse models, rD/OK-AL was attenuated after intranasal inoculation, leading to high antibody levels against IDV. Post-challenge with wild-type virus, IDV was undetectable in respiratory tissues, indicating complete protection. These results suggest that rD/OK-AL could be a promising candidate for developing live attenuated vaccines to control BRDC [12, 102, 92, 2, 26, 28, 103, 31]. The research investigates the immunogenicity and efficacy of a quadrivalent neuraminidase RNA particle vaccine, Sequivity NA, marketed under the brand name Sequivity® IAV-S NA. This vaccine uses four neuraminidase antigens representing the predominant NA clades of Influenza A virus (IAV) subtypes H1N1, H1N2, and H3N2 prevalent in swine populations across the United States. During the trials, pigs received two vaccinations at three days and three weeks of age, followed by challenges with either homologous or heterologous IAV strains. The vaccine successfully elicited a strong serum neuraminidase inhibition (NI) antibody response. It conferred protection against both homologous and heterologous IAV-S strains, as in 2023, Kitikoon et al. [104] stated: "Quadrivalent neuraminidase RNA particle vaccine protects pigs against homologous and heterologous strains of swine influenza virus infection” (Figure 3) [104, 105, 106, 107, 66, 68, 108, 109, 48].
Moreover, Bovine Respiratory Syncytial Virus (BRSV) and Bovine Parainfluenza 3 Virus (BPIV3) are significant pathogens in bovine respiratory disease (BRD), leading to substantial morbidity and economic losses. Their intricate interaction with the host's immune system necessitates the presence of neutralizing antibodies and cell-mediated immunity. According to Makoschey and Berge (2021), combination vaccines targeting both viruses are commonly employed, and understanding their roles within the BRD complex can facilitate the development of effective vaccines [110, 67, 111, 26, 112, 113, 30, 114]. Moreover, research uncovers three newly identified triple-reassortant H1N1 swine influenza viruses from pigs in Tianjin, Northern China. In 2016, Sun et al. [90] stated that "these viruses possess a genetic composition derived from the 2009 pandemic H1N1 strain, Eurasian swine, and triple-reassortant swine lineages". The reassortment occurring in Tianjin presents a potential risk to human health, given that swine influenza, a highly contagious respiratory infection, has led to outbreaks in both the United States and China [115, 20, 67, 80]. Similarly, the Influenza D virus has emerged as a novel contributor to bovine respiratory disease (BRD), leading to notable production and economic setbacks in feedlot operations. As in 2020, Alvarez et al. [116] noted, "IDV infection is endemic in La Pampa, with 68% of the examined samples testing positive." However, the clinical significance of the virus in Argentina remains to be thoroughly investigated [116, 117]. In the same way, a novel influenza virus of genus D has been identified in pigs and cattle in the United States and France, showing a genome similarity of 94% to 99% to its U.S. counterpart. As in 2015, Ducatez et al. [118] state, "The virus, designated C/swine/Oklahoma/1334/2011 (C/OK), replicates in ferrets, which are the preferred animal model for studying influenza in humans." [118, 116, 119, 41, 117]. In Luxembourg, the influenza D virus (IDV) is widespread among cattle farms, with antibodies identified in 80.2% of cattle samples collected in 2016. The seroprevalence of the virus rose significantly from 0% to 5.9% between 2012 and 2015 [120]. The United States revealed that 19.1% of 256 swine tested were seropositive for influenza D virus, with an overall IDV seropositivity rate of 42.7% [121]. Moreover, the Influenza D virus predominantly occurs in cattle across numerous countries, exhibiting a notably high seropositivity rate of 77.5% in the United States. The virus is associated with mild to moderate respiratory ailments. It plays a role in the bovine respiratory disease complex (BRDC), representing the most economically burdensome illness impacting the U.S. cattle industry [122, 28]. The study assessed antibody levels against influenza viruses across seven age cohorts during Poland's 2018/2019 epidemic season. The peak antibody response was observed in the 10-14 age group for the A/Singapore/INFIMH-16-0019/2016 (H3N2) and B/Phuket/3073/2013 Yamagata lineage antigens. Influenza represents a significant risk to vulnerable populations, including individuals over 65, children under two years, pregnant women, and those with chronic health conditions [123, 105, 107]. A novel strain of the Influenza D virus has been isolated from affected cattle in Japan and is linked to respiratory illnesses. The hemagglutinin-esterase-fusion (HEF) gene does not belong to any recognized phylogenetic lineages [125, 11, 14]. At the same time, Tropheryma whipplei pneumonia has been associated with HIV-2 infection in an individual with the virus. Dogs can transmit various pathogens to humans, including canine influenza A and prevalent human influenza viruses. Italy revealed a low prevalence of exposure to influenza D virus among adult household dogs, showing no correlation between IDV infection and clinical manifestations in dogs, as well as a lack of cross-reactivity between IDV and human influenza C virus (ICV) [50, 27, 87]. A study published in Emerging Microbes & Infections investigated the virulence and transmissibility of canine H3N2 influenza viruses (CIVs) isolated from dogs in South Korea between 2009 and 2013. The analysis revealed that these viruses exhibited a close genetic relationship with strains previously identified in dogs from both Korea and China. Notably, the research identified non-synonymous mutations within the canine viruses, particularly affecting the presumed H3 antigenic sites, the neuraminidase (NA) stalk regions, and various internal genes. Furthermore, the study elucidated the genetic contributions of the NA, nucleoprotein (NP), and matrix (M) genes to the adaptability of CIVs that originated from the avian H3N2 virus [126, 106, 107, 69, 29].
Likewise, the virosomal influenza vaccine administered to pigs exhibited no significant lesions in the lung, spleen, liver, or kidney tissue samples. Nevertheless, follicular lymphoid hyperplasia was detected in 71.87% of the pigs in the G2 group, while mild histiocytic infiltration was noted in 11.11% of the pigs from the G1 group and 15.62% from the G2 group. The polyvalent influenza virosome vaccine demonstrated a commendable safety profile and non-cytotoxicity in pigs, as evidenced by the findings from the TUNEL assay and the absence of adverse reactions following vaccination. Furthermore, in (2023), Haach et al. [127] indicated that “the follicular lymphoid hyperplasia observed in the mediastinal lymph nodes of vaccinated pigs remains to be investigated” [127, 73]. A DNA vaccine, FluD-Vax, has been engineered to safeguard guinea pigs from infection by two distinct lineages of the influenza D virus (D/OK and D/660). This vaccine expresses the consensus hemagglutinin-esterase fusion protein (HEF) and has undergone testing against these lineages in guinea pigs. The vaccine elicited substantial levels of neutralizing antibodies against representatives of the influenza D virus lineages, specifically D/OK and D/660 [128, 42, 129]. The study investigates the innate immune response within the respiratory epithelium of human, porcine, and bovine hosts during influenza virus infection to uncover potential factors influencing viral cross-species transmission [131]. Influenza C and D viruses exhibit genetic similarities and utilize 9-O-acetylated sialic acids for cellular entry. Both viruses target ciliated cells, operating independently of the host and ambient temperature. Temperature pronounced affects influenza C virus (ICV) replication in cultured environments, whereas influenza D virus demonstrates efficient replication regardless of temperature and host species [131, 132, 133, 134]. Influenza viruses, encompassing types A, B, C, and D (IAV, IBV, ICV, and IDV), impact various animal species, including humans. The non-structural protein 1 (NS1) is a multifunctional protein in all influenza types, undermining host antiviral defenses. Despite performing analogous functions, NS1 proteins from various types exhibit minimal amino acid sequence homology [135, 13]. Moreover, in contrast to that for prevention, Wild watermelon, a plant rich in phytochemicals and phytoestrogens, has emerged as a promising functional food in the fight against influenza. The plant's phytochemicals and phytoestrogens, which include polyphenols, flavonoids, and prenylated compounds, demonstrate the ability to inhibit viral growth, entry, and replication. This study emphasizes the potential of wild watermelon as a preventive and therapeutic agent against influenza, exhibiting notable antiviral efficacy. Influenza, a zoonotic disease with significant public health implications, is marked by antigenic mutations that affect transmissibility and drug resistance. While antiviral medications have been developed to target various stages of viral replication, resistance can develop within a timeframe ranging from years to decades [136, 137]. It has seven RNA segments and one major surface glycoprotein, the hemagglutinin-esterase-fusion. The virus has been identified in several countries, including France, Italy, Luxembourg, Canada, Mexico, China, Mississippi, Japan, and Nebraska. Notably, the virus has been detected in cattle, sheep, swine, horses, and goats. However, its viral genome has been confirmed only in certain animal species, with specific antibodies detected in others. In 2022, a study conducted by Trombetta et al. [138] to evaluate the prevalence of antibodies against two IDV lineages (D/660 and D/OK) in archived serum samples from swine veterinarians in Italy collected in 2004, results indicated that cattle may come into contact with the virus either during transportation or immediately afterward [138, 139]. At the same time, there is research to assess the extent of maternally acquired passive immunity in cattle from Mississippi and its influence on the onset of infectious diseases. Among 55 ailing calves, 32.7% exhibited seropositivity for Influenza D Virus (IDV), with a geometric mean viral titer (GMT) of 172.8 (±7215.7). These calves had been housed at the facility for an average duration of 24 days at the time of sampling and had undergone 1.9 treatments for respiratory illness. Quantitative RT-PCR analysis of respiratory swabs identified IDV in 29.1% (55 out of 189) of the sick calves and 2.4% (2 out of 82) of the healthy calves, supporting the hypothesis that IDV infection correlates with bovine respiratory disease (BRD) in dairy cattle. In 2015, Ferguson et al. [140] state, “Influenza D virus infection was observed in Mississippi beef cattle with a significant percentage showing seropositivity.” The calves testing positive for IDV had been at the facility for an average of 19.4 days, implying that active infection may develop within the first week of arrival at the order-buying facility. Two healthy calves, who had only been at the facility for seven days, shared the same pen. BRD is a multifaceted, multi-pathogen disease with various contributing agents. IDV, detected in cattle displaying respiratory symptoms, could potentially be one of the multiple pathogens that compromise host immunity and exacerbate the pathogenesis of respiratory disease in cattle. Metagenomics analysis of respiratory samples from 50 dairy calves with BRD revealed contig reads for bovine adenovirus 3, bovine rhinotracheitis virus A, and IDV in 62% of the specimens. However, other respiratory pathogens might account for the elevated IDV prevalence in sick versus healthy herds. The findings indicated that 94% of neonatal cattle sampled between 2013 and 2014 acquired immunity against IDV through maternal colostrum, though this immunity appeared to wane with age. By six months, many cattle were likely vulnerable to IDV infection [140, 141].
Efficacy of Two Intranasal Vaccines in Mitigating Bovine Respiratory Disease among Neonatal Beef Calves. Bovine respiratory syncytial virus (BRSV) and bovine parainfluenza-3 virus (bPI3V) are significant contributors to bovine respiratory disease (BRD) in neonatal calves globally. Vaccination is extensively implemented to mitigate BRD, with the development of intranasal vaccines for BRSV and bPI3V aimed at circumventing the interference posed by maternally derived antibodies. Numerous experimental challenge trials have demonstrated the efficacy of these intranasal vaccines; however, their effectiveness in practical field settings, particularly among newborn beef calves, has been less frequently validated. This field trial evaluated the comparative effectiveness of a commercially launched commercial BRSV-bPI3V intranasal vaccine against a benchmark counterpart in newborn beef calves raised within a cow-calf management system. A total of 935 calves across 39 farms were randomized into two vaccination cohorts (Bovalto et al. [Vaccine A], n = 468; Rispoval RS + PI3 Intranasal [Vaccine B], n = 467) and closely monitored throughout the in-house risk period, extending up to three months post-vaccination. A non-inferiority analysis assessed the disparity in BRD prevalence between the two vaccine groups. No statistically significant differences were identified between the vaccines concerning clinical outcomes such as morbidity, mortality, the interval between vaccination and BRD onset, and the treatments administered. Given that the upper limit of the two-sided 95% confidence interval for the difference in BRD prevalence between the treatment groups (0.8%) remained below the non-inferiority margin (d = 5%), Vaccine A was deemed non-inferior [142, 122, 113].
Table 2. Reactivity profile of mouse antisera against various viral strains. |
||||||
Antiserum |
Test |
Titer against OK |
Titer against NE |
Titer against Y16 |
Titer against Y19 |
Titer against rCA/OK |
αOK |
HI |
5,120 |
1,280 |
1,280 |
640 |
640 |
|
VN |
12,800 |
6,400 |
3,200 |
1,600 |
1,600 |
αNE |
HI |
80 |
640 |
320 |
160 |
80 |
|
VN |
100 |
3,200 |
6,400 |
800 |
200 |
αY16 |
HI |
320 |
320 |
640 |
80 |
80 |
|
VN |
800 |
800 |
3,200 |
800 |
200 |
αY19 |
HI |
640 |
160 |
320 |
640 |
320 |
|
VN |
800 |
800 |
1,600 |
1,600 |
80 |
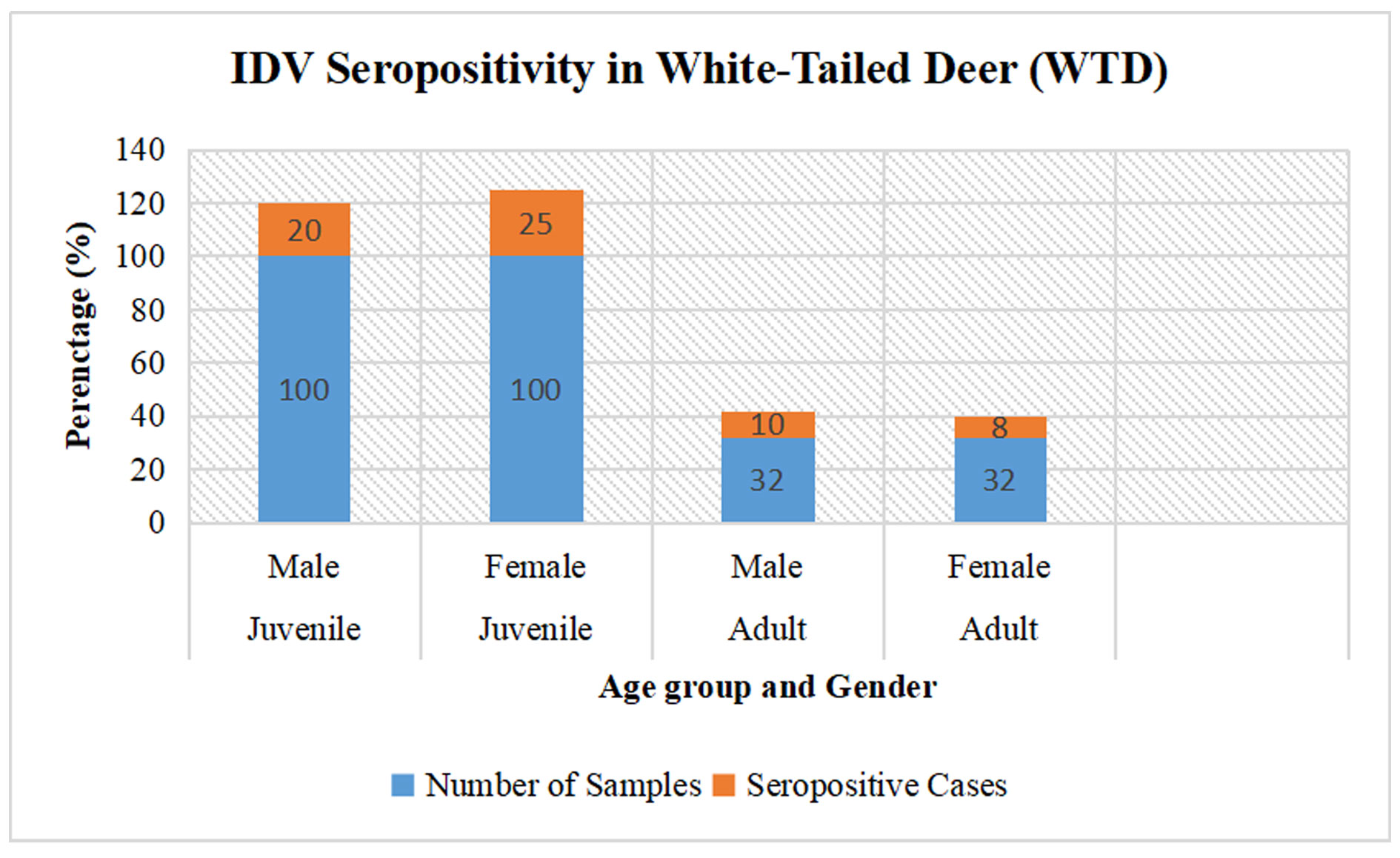
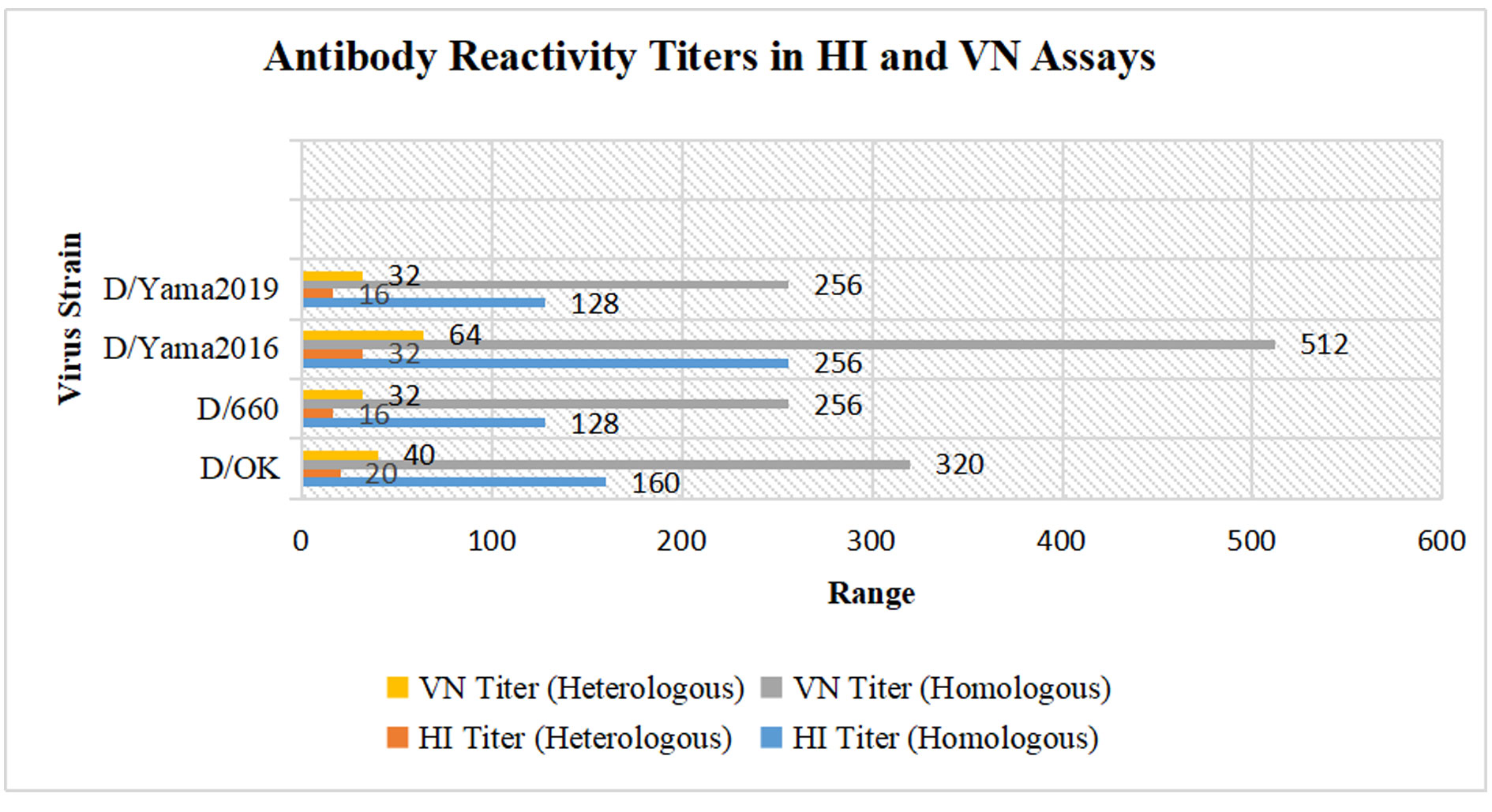
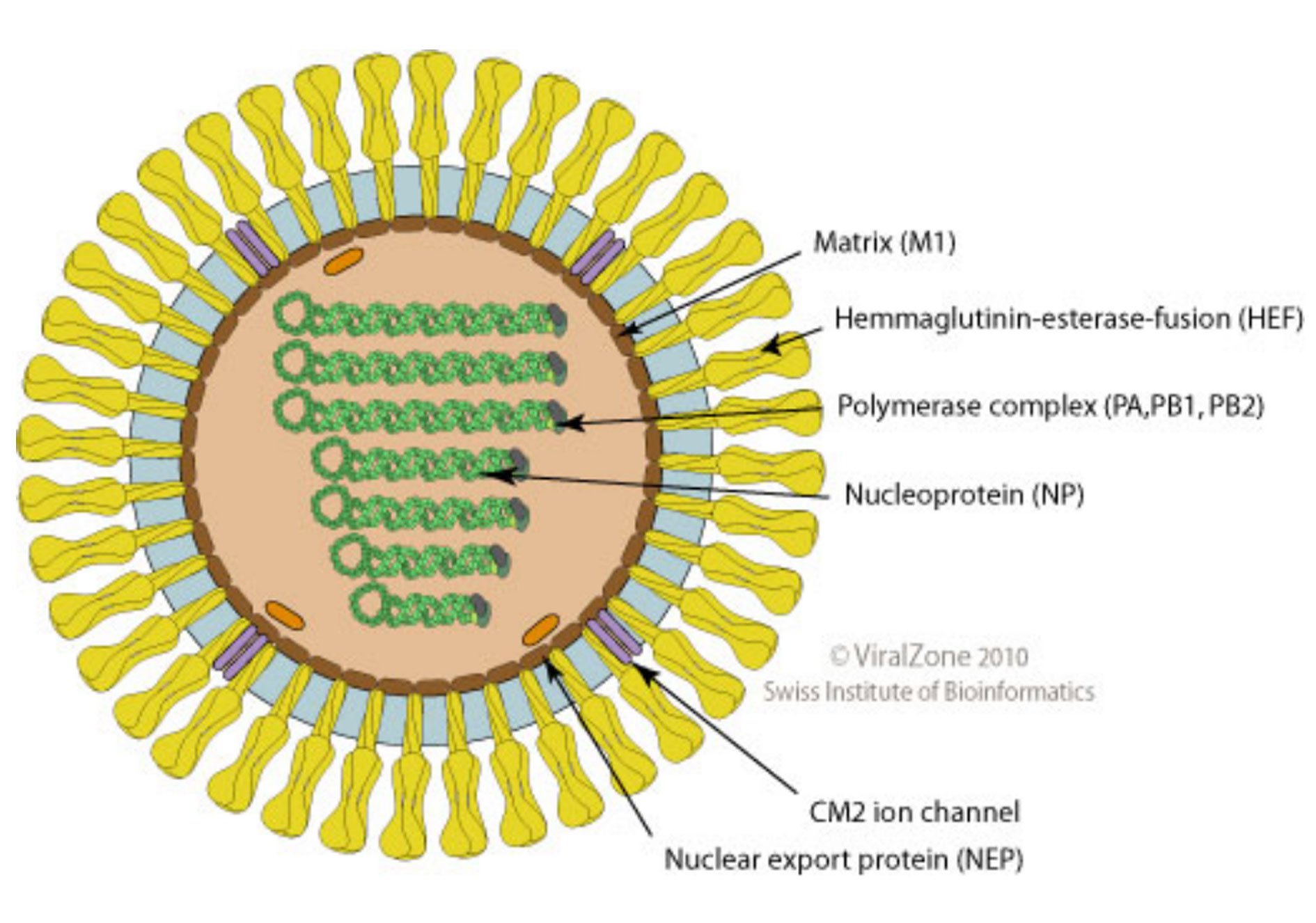
The H1N1pdm09 virus has been transmitted from humans to swine in Brazil, with at least 30 distinct introductions occurring between 2009 and 2011 [149]. These introductions are likely characterized by self-limited infections in swine, resulting in minimal or negligible onward transmission. Nevertheless, the virus persisted in certain instances. It continued to propagate successfully among swine, as demonstrated by the clustering of monophyletic clades that encompass three or more swine sequences isolated in Brazil over several years. The HA and NA sequences from swine collected in Brazil exhibited elongated branch lengths, indicative of substantial genetic divergence and limited sampling [149]. Moreover, the D/Yama2019 lineage-like Influenza D virus (IDV) has been detected in cattle in China. The study revealed the identification of an IDV strain with nearly complete genomic sequences within Chinese cattle herds, demonstrating genetic proximity to strains found in Japan while exhibiting significant divergence from previously reported Chinese IDV strains. This finding underscores the necessity for enhanced surveillance of D/Yama2019-like viruses to gain deeper insights into the epidemiology and diversity of IDV in China [150]. The Influenza D virus is a multi-host pathogen predominantly residing in bovine populations. It specifically attaches to 9-O-acetylated N-acetylneuraminic acid, which confers a selective evolutionary advantage, facilitating an expanded range of potential hosts [151, 152].
Influenza C and D viruses displayed a unique respiratory tissue tropism in pathogenesis in guinea pigs. Community-acquired pneumonia (CAP) in children exhibits a severity greater than that of the influenza B virus yet comparable to CAP associated with the influenza A virus. This investigation aimed to elucidate the replication dynamics, tissue tropism, and pathogenesis of human ICV (huICV) about the swine influenza D virus (swIDV) in guinea pigs. Intranasal inoculation of both viruses did not elicit clinical symptoms; however, the infected subjects shed the virus in nasal washes. The huICV demonstrated replication in the nasal turbinates, soft palate, and trachea but not in the pulmonary tissues, whereas the sword was capable of replicating across all four examined tissues [153, 133, 20, 154, 63, 129, 130]. The Influenza D virus (IDV), a member of the Orthomyxoviridae family, has been identified in various regions, including North America, Europe, Asia, and Africa. In South Korea, the inaugural report regarding the detection of viral RNA and the seroprevalence of IDV was conducted in 2022. Viral RNA was analyzed using real-time RT-PCR assays on 999 nasal swabs and lung tissues from cattle and 2,391 from pigs. The seroprevalence of IDV was assessed by testing 742 cattle and 1,627 pig sera utilizing a hemagglutination inhibition (HI) assay. The positive rate for viral RNA was 1.4% in cattle, while no viral RNA was identified in pigs. The study revealed a viral RNA positivity rate of 1.4% among cattle, with seropositivity rates recorded at 54.7% for cattle and 1.4% for pigs. The geometric mean antibody titers (GMT) were 68.3 in cattle and 48.5 in pigs. This study marks the first documentation of both viral RNA and antibodies to IDV in the Republic of Korea. IDV can infect and disseminate among a broad spectrum of hosts, including domestic cattle, pigs, sheep, goats, horses, camels, poultry, and wild species such as feral pigs. Cattle are recognized as the principal reservoir for IDV, with infections linked to mild to severe respiratory clinical manifestations. The recently identified IDV is believed to primarily cause respiratory illness in cattle, with significantly lesser effects on pigs. In 2022, Korean native and non-indigenous beef cattle constituted 90% of the national cattle population, while dairy cattle accounted for merely 10%. The relatively small proportion of dairy cattle in the national herd can be attributed to the lesser significance of dairy products in the traditional Korean diet [155, 117].
Clinical Manifestations, Diagnosis, and Prospective Vaccines.Influenza D virus is transmitted via direct contact and aerosolized particles over short distances. The majority of available data regarding this disease's clinical manifestations pertain predominantly to cattle infections. Notably, the potential existence of asymptomatic carriers has also been documented in bovine and various other animal species.
1. Cattle: Cattle serve as the primary reservoir for IDV, with extensive serological studies confirming high seroprevalence across North America and Europe and evidence of the virus's potential contribution to BRD.
2. Swine: IDV circulation has been confirmed in swine populations in several countries, but seroprevalence is generally lower than in cattle, suggesting further investigation into pigs' roles in IDV ecology is warranted.
3. Camels: High seroprevalence of IDV has been observed in camels from Africa and Asia, indicating they may also act as significant reservoirs for the virus.
4. Small Ruminants: Sheep and goats exhibit lower IDV susceptibility than cattle and camels, with varying seroprevalence observed in North America and Africa.
5. Horses: Horses show some seroprevalence to IDV, indicating potential interspecies transmission, although the virus does not appear to cause respiratory disease in equines.
6. Wild Animals: Feral swine and wild deer in North America exhibit varying seroprevalence to IDV, suggesting a possible role in the virus's ecology and transmission between domestic and wild species (Figuure 4) [156, 39].
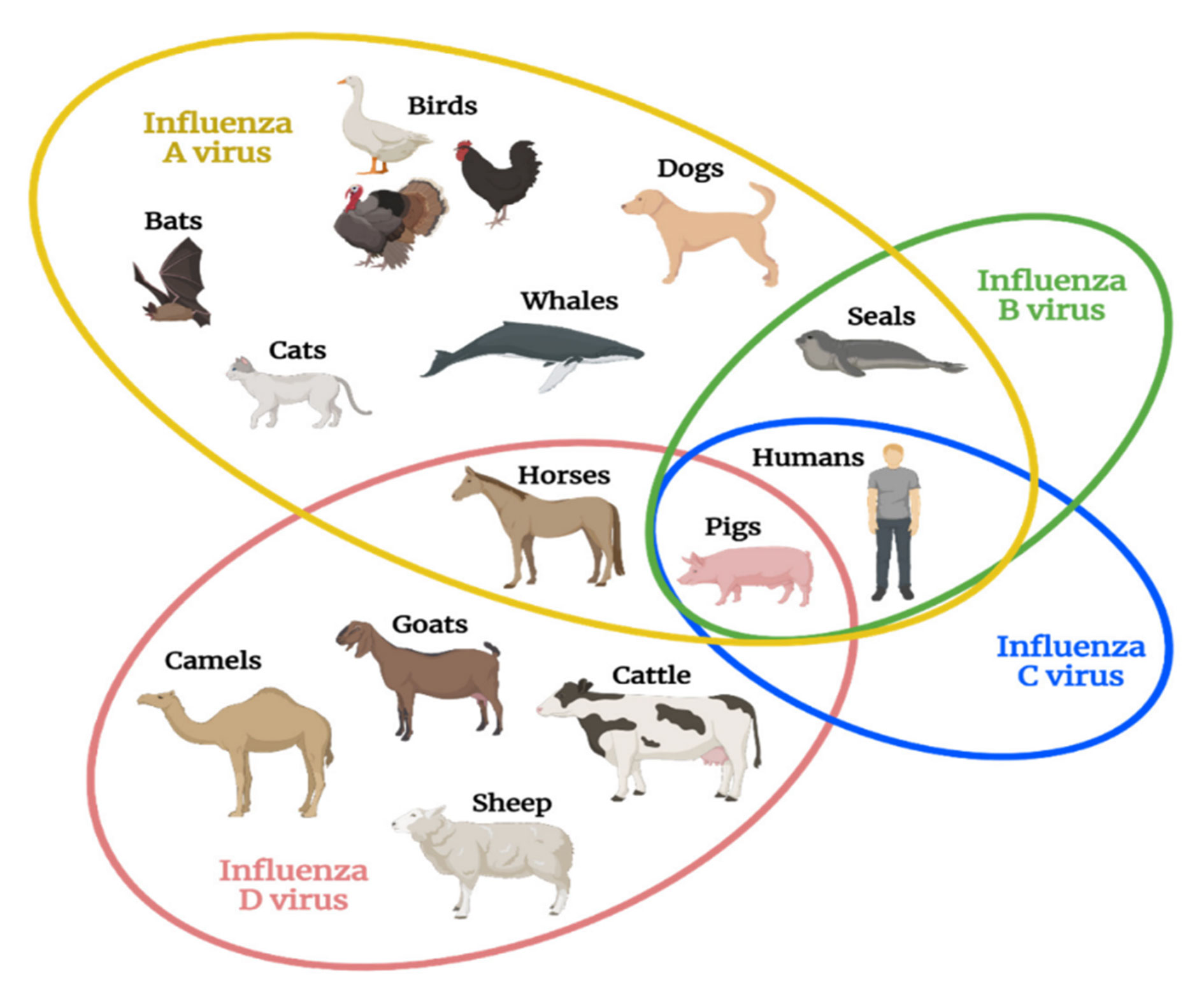
Approaches for Controlling IDV Infections and Addressing Future Risks. Influenza viruses have caused significant mortality through annual epidemics and pandemics, prompting advancements in public health infrastructure and surveillance. Recent studies have developed effective diagnostics for the Influenza D virus (IDV), including a sensitive real-time RT-PCR assay and a multiplex assay that detects multiple respiratory pathogens. Additionally, bioaerosol surveillance has shown promise for monitoring IDV in public areas. Innovative vaccine strategies, such as a DNA vaccine targeting IDV lineages and an inactivated virus vaccine, have demonstrated protective efficacy in animal models. These vaccines resulted in significant antibody responses and reduced viral titers. While progress has been made in managing IDV infections, further research is essential to evaluate and enhance antiviral strategies to prevent future outbreaks and zoonotic transmission to humans [25, 159, 38].
No applicable.
Ethics approval
No applicable.
Data availability
The data will be available upon request.
Funding
None.
Authors’ contribution
Abdul Bari Hejran undertook the responsibilities of data collection and analysis, as well as the initial drafting of the manuscript and the meticulous review of the methodology. Khaidarov Saken spearheaded the conceptualization and design of the study, orchestrated the research activities, contributed to the drafting and revision of the manuscript, and granted approval for the final version prior to submission. Parwiz Niazi executed a comprehensive literature review, facilitated data interpretation, and contributed to the formulation of specific sections of the manuscript. Rahmatullah Afghan rendered assistance with statistical analysis, enriched the interpretation of findings, and provided critical revisions to the manuscript content. Each author played a pivotal role in ensuring the meticulousness and rigor of the study from its inception to final submission.
Competing interests
None.
- Liu R, Sheng Z, Huang C, Wang D, Li F: Influenza D virus. Curr Opin Virol 2020, 44: 154-161.
- Su S, Fu X, Li G, Kerlin F, Veit M: Novel Influenza D virus: Epidemiology, pathology, evolution and biological characteristics. Virulence 2017, 8(8): 1580-1591.
- Wang P, Zheng M, Lau SY, Chen P, Mok BW, Liu S, Liu H, Huang X, Cremin CJ, Song W, et al: Generation of DelNS1 Influenza Viruses: a Strategy for Optimizing Live Attenuated Influenza Vaccines. mBio 2019, 10(5): e02180-19.
- Zhang X, Ross TM: Anti-neuraminidase immunity in the combat against influenza. Expert Rev Vaccines 2024, 23(1): 474-484.
- Kondratiuk K, Poznańska A, Szymański K, Czajkowska E, Mańkowski B, Brydak LB: Occurrence of Circulating Antibodies against the Hemagglutinins of Influenza Viruses in the 2022/2023 Epidemic Season in Poland. Viruses 2024, 16(7): 1105.
- Ferguson L, Olivier AK, Genova S, Epperson WB, Smith DR, Schneider L, Barton K, McCuan K, Webby RJ, Wan XF: Pathogenesis of Influenza D Virus in Cattle. J Virol 2016, 90(12): 5636-5642.
- Jiang WM, Wang SC, Peng C, Yu JM, Zhuang QY, Hou GY, Liu S, Li JP, Chen JM: Identification of a potential novel type of influenza virus in Bovine in China. Virus Genes 2014, 49(3): 493-496.
- Lutteke N, Sausy A, Black AP, Wildschutz F, Muller CP: Neutralizing antibodies against influenza A in pigs before and after the 2009 pandemic, Luxembourg. Vet Microbiol 2014, 169(1-2): 96-101.
- Chiapponi C, Faccini S, De Mattia A, Baioni L, Barbieri I, Rosignoli C, Nigrelli A, Foni E: Detection of Influenza D Virus among Swine and Cattle, Italy. Emerg Infect Dis 2016, 22(2): 352-354.
- Molini U, Curini V, Jacobs E, Tongo E, Berjaoui S, Hemberger MY, Puglia I, Jago M, Khaiseb S, Cattoli G, et al: First influenza D virus full-genome sequence retrieved from livestock in Namibia, Africa. Acta Trop 2022, 232: 106482.
- Wang M, Veit M: Hemagglutinin-esterase-fusion (HEF) protein of influenza C virus. Protein Cell 2016, 7(1): 28-45.
- Ishida H, Murakami S, Kamiki H, Matsugo H, Katayama M, Sekine W, Ohira K, Takenaka-Uema A, Horimoto T: Generation of a recombinant temperature-sensitive influenza D virus. Sci Rep 2023, 13(1): 3806.
- Yu J, Huang C, Sheng Z, Wang Z, Li F, Wang D: Identification of One Critical Amino Acid Residue of the Nucleoprotein as a Determinant for In Vitro Replication Fitness of Influenza D Virus. J Virol 2021, 95(18): e0097121.
- Yu J, Hika B, Liu R, Sheng Z, Hause BM, Li F, Wang D: The Hemagglutinin-Esterase Fusion Glycoprotein Is a Primary Determinant of the Exceptional Thermal and Acid Stability of Influenza D Virus. mSphere 2017, 2(4) e00254-17.
- Limaye S, Lohar T, Dube H, Ramasamy S, Kale M, Kulkarni-Kale U, Kuchipudi SV: Rapid evolution leads to extensive genetic diversification of cattle flu Influenza D virus. Commun Biol 2024, 7(1): 1276.
- Huang C, Yu J, Hause BM, Park JY, Sreenivasan C, Uprety T, Sheng Z, Wang D, Li F: Emergence of new phylogenetic lineage of Influenza D virus with broad antigenicity in California, United States. Emerg Microbes Infect 2021, 10(1): 739-742.
- Saito T, Sakuma S, Mine J, Uchida Y, Hangalapura BN: Genetic Diversity of the Hemagglutinin Genes of Influenza a Virus in Asian Swine Populations. Viruses 2022, 14(4): 747.
- Limaye S, Shelke A, Kale MM, Kulkarni-Kale U, Kuchipudi SV: IDV Typer: An Automated Tool for Lineage Typing of Influenza D Viruses Based on Return Time Distribution. Viruses 2024, 16(3): 373.
- Song H, Gao GF: Evaluation of the Glycan-Binding and Esterase Activities of Hemagglutinin-Esterase-Fusion Glycoprotein from Influenza D Virus. Methods Mol Biol 2022, 2556: 187-203.
- Song H, Qi J, Khedri Z, Diaz S, Yu H, Chen X, Varki A, Shi Y, Gao GF: An Open Receptor-Binding Cavity of Hemagglutinin-Esterase-Fusion Glycoprotein from Newly-Identified Influenza D Virus: Basis for Its Broad Cell Tropism. PLoS Pathog 2016, 12(1): e1005411.
- Kim H, Webster RG, Webby RJ: Influenza Virus: Dealing with a Drifting and Shifting Pathogen. Viral Immunol 2018, 31(2): 174-183.
- Huang ZZ, Tan J, Huang P, Li BS, Guo Q, Liang LJ: The evolutionary features and roles of single nucleotide variants and charged amino acid mutations in influenza outbreaks during NPI period. Sci Rep 2024, 14(1): 20418.
- Nemanichvili N, Berends AJ, Tomris I, Barnard KN, Parrish CR, Gröne A, Rijks JM, Verheije MH, de Vries RP: Influenza D binding properties vary amongst the two major virus clades and wildlife species. Vet Microbiol 2022, 264: 109298.
- Kaplan BS, Falkenberg S, Dassanayake R, Neill J, Velayudhan B, Li F, Vincent AL: Virus strain influenced the interspecies transmission of influenza D virus between calves and pigs. Transbound Emerg Dis 2021, 68(6): 3396-3404.
- Asha K, Kumar B: Emerging Influenza D Virus Threat: What We Know so Far! J Clin Med 2019, 8(2): 192.
- Nissly RH, Zaman N, Ibrahim PAS, McDaniel K, Lim L, Kiser JN, Bird I, Chothe SK, Bhushan GL, Vandegrift K, et al: Influenza C and D viral load in cattle correlates with bovine respiratory disease (BRD): Emerging role of orthomyxoviruses in the pathogenesis of BRD. Virology 2020, 551: 10-15.
- Sheng Z, Ran Z, Wang D, Hoppe AD, Simonson R, Chakravarty S, Hause BM, Li F: Genomic and evolutionary characterization of a novel influenza-C-like virus from swine. Arch Virol 2014, 159(2): 249-255.
- Uprety T, Sreenivasan CC, Thomas M, Hause B, Christopher-Hennings J, Miskimis D, Pillatzki A, Nelson E, Wang D, Li F: Prevalence and characterization of seven-segmented influenza viruses in bovine respiratory disease complex. Virology 2023, 587: 109859.
- Lee J, Wang L, Palinski R, Walsh T, He D, Li Y, Wu R, Lang Y, Sunwoo SY, Richt JA, et al: Comparison of Pathogenicity and Transmissibility of Influenza B and D Viruses in Pigs. Viruses 2019, 11(10): 905.
- Holwerda M, Laloli L, Wider M, Schönecker L, Becker J, Meylan M, Dijkman R: Establishment of a Reverse Genetic System from a Bovine Derived Influenza D Virus Isolate. Viruses 2021, 13(3): 502.
- Shi HF, Zhu YM, Yan H, Ma L, Wang XZ, Xue F: Pathogenesis of a Chinese strain of bovine adenovirus type 3 infection in albino guinea pigs. Arch Virol 2014, 159(12): 3211-3218.
- Yu J, Li F, Wang D: The first decade of research advances in influenza D virus. J Gen Virol 2021, 102(1): jgv001529.
- Rijal P, Wang BB, Tan TK, Schimanski L, Janesch P, Dong T, McCauley JW, Daniels RS, Townsend AR, Huang KA: Broadly Inhibiting Antineuraminidase Monoclonal Antibodies Induced by Trivalent Influenza Vaccine and H7N9 Infection in Humans. J Virol 2020, 94(4): e01182-19.
- Oliva J, Eichenbaum A, Belin J, Gaudino M, Guillotin J, Alzieu JP, Nicollet P, Brugidou R, Gueneau E, Michel E, et al: Serological Evidence of Influenza D Virus Circulation Among Cattle and Small Ruminants in France. Viruses 2019, 11(6): 516.
- Fusade-Boyer M, Pato PS, Komlan M, Dogno K, Batawui K, Go-Maro E, McKenzie P, Guinat C, Secula A, Paul M, et al: Risk Mapping of Influenza D Virus Occurrence in Ruminants and Swine in Togo Using a Spatial Multicriteria Decision Analysis Approach. Viruses 2020, 12(2): 128.
- Skelton RM, Huber VC: Comparing Influenza Virus Biology for Understanding Influenza D Virus. Viruses 2022, 14(5): 1036.
- Ruiz M, Puig A, Bassols M, Fraile L, Armengol R: Influenza D Virus: A Review and Update of Its Role in Bovine Respiratory Syndrome. Viruses 2022, 14(12): 2717.
- Abdelwhab EM, Mettenleiter TC: Zoonotic Animal Influenza Virus and Potential Mixing Vessel Hosts. Viruses 2023, 15(4): 980.
- Hanon JB, Cay B: Seroprevalence of pestivirus infections is low in Belgian small ruminant flocks and is significantly associated with the presence of cattle. Prev Vet Med 2021, 195: 105446.
- Kondratiuk K, Hallmann E, Szymański K, Łuniewska K, Poznańska A, Brydak LB: Prevalence of circulating antibodies against hemagglutinin of influenza viruses in epidemic season 2021/2022 in Poland. Acta Biochim Pol 2024, 71: 12289.
- Hause BM, Collin EA, Liu R, Huang B, Sheng Z, Lu W, Wang D, Nelson EA, Li F: Characterization of a novel influenza virus in cattle and Swine: proposal for a new genus in the Orthomyxoviridae family. mBio 2014, 5(2): e00031-00014.
- Collin EA, Sheng Z, Lang Y, Ma W, Hause BM, Li F: Cocirculation of two distinct genetic and antigenic lineages of proposed influenza D virus in cattle. J Virol 2015, 89(2): 1036-1042.
- Zhang T, Yin C, Boyd DF, Quarato G, Ingram JP, Shubina M, Ragan KB, Ishizuka T, Crawford JC, Tummers B, et al: Influenza Virus Z-RNAs Induce ZBP1-Mediated Necroptosis. Cell 2020, 180(6): 1115-1129.e1113.
- Trombetta CM, Marchi S, Manini I, Kistner O, Li F, Piu P, Manenti A, Biuso F, Sreenivasan C, Druce J, et al: Influenza D Virus: Serological Evidence in the Italian Population from 2005 to 2017. Viruses 2019, 12(1): 30.
- Zhai SL, Zhang H, Chen SN, Zhou X, Lin T, Liu R, Lv DH, Wen XH, Wei WK, Wang D, et al: Influenza D Virus in Animal Species in Guangdong Province, Southern China. Emerg Infect Dis 2017, 23(8): 1392-1396.
- Carascal MB, Pavon RDN, Rivera WL: Recent Progress in Recombinant Influenza Vaccine Development Toward Heterosubtypic Immune Response. Front Immunol 2022, 13: 878943.
- Zhou X, Li N, Luo Y, Liu Y, Miao F, Chen T, Zhang S, Cao P, Li X, Tian K, et al: Emergence of African Swine Fever in China, 2018. Transbound Emerg Dis 2018, 65(6): 1482-1484.
- Wang G, Dos Anjos Borges LG, Stadlbauer D, Ramos I, Bermúdez González MC, He J, Ding Y, Wei Z, Ouyang K, Huang W, et al: Characterization of swine-origin H1N1 canine influenza viruses. Emerg Microbes Infect 2019, 8(1): 1017-1026.
- Olsen SJ, Winn AK, Budd AP, Prill MM, Steel J, Midgley CM, Kniss K, Burns E, Rowe T, Foust A, et al: Changes in influenza and other respiratory virus activity during the COVID-19 pandemic-United States, 2020-2021. Am J Transplant 2021, 21(10): 3481-3486.
- Trombetta CM, Marchi S, Marotta MG, Moreno A, Chiapponi C, Montomoli E, Lanave G, Camero M, Martella V: Detection of Influenza D Antibodies in Dogs, Apulia Region, Italy, 2016 and 2023. Emerg Infect Dis 2024, 30(5): 1045-1047.
- Trombetta CM, Marchi S, Manini I, Kistner O, Li F, Piu P, Manenti A, Biuso F, Sreenivasan C, Druce J, et al: Influenza D Virus: A Potential Threat for Humans? Proceedings 2020, 50(1): 36.
- Lee E, Kim EJ, Kim BH, Song JY, Cho IS, Shin YK: Molecular analyses of H3N2 canine influenza viruses isolated from Korea during 2013-2014. Virus Genes 2016, 52(2): 204-217.
- Yang JR, Cheng CY, Chen CY, Lin CH, Kuo CY, Huang HY, Wu FT, Yang YC, Wu CY, Liu MT, et al: A virus-like particle vaccination strategy expands its tolerance to H3N2 antigenic drift by enhancing neutralizing antibodies against hemagglutinin stalk. Antiviral Res 2017, 140(1): 62-75.
- Mossad SB: Hey, Doc: Could the 2023-2024 cold and flu season finally be the calm after the storm? Cleve Clin J Med 2023, 90(12): 729-734.
- Zhu S, Quint J, León TM, Sun M, Li NJ, Tenforde MW, Jain S, Schechter R, Hoover C, Murray EL: Interim Influenza Vaccine Effectiveness Against Laboratory-Confirmed Influenza - California, October 2023-January 2024. MMWR Morb Mortal Wkly Rep 2024, 73(8): 175-179.
- Guan M, Jacobson O, Sarafianos G, Baroch J, Deliberto TJ, Wan XF: Exposure of white-tailed deer in North America to influenza D virus. Virology 2022, 573: 111-117.
- Strässle M, Laloli L, Gultom M, V'Kovski P, Stoffel MH, Crespo Pomar S, Chanfon Bätzner A, Ebert N, Labroussaa F, Dijkman R, et al: Establishment of caprine airway epithelial cells grown in an air-liquid interface system to study caprine respiratory viruses and bacteria. Vet Microbiol 2021, 257: 109067.
- Lion A, Secula A, Rançon C, Boulesteix O, Pinard A, Deslis A, Hägglund S, Salem E, Cassard H, Näslund K, et al: Enhanced Pathogenesis Caused by Influenza D Virus and Mycoplasma bovis Coinfection in Calves: a Disease Severity Linked with Overexpression of IFN-γ as a Key Player of the Enhanced Innate Immune Response in Lungs. Microbiol Spectr 2021, 9(3): e0169021.
- Gaudino M, Lion A, Sagné E, Nagamine B, Oliva J, Terrier O, Errazuriz-Cerda E, Scribe A, Sikht FZ, Simon E, et al: The Activation of the RIG-I/MDA5 Signaling Pathway upon Influenza D Virus Infection Impairs the Pulmonary Proinflammatory Response Triggered by Mycoplasma bovis Superinfection. J Virol 2023, 97(2): e0142322.
- Guan M, Blackmon S, Olivier AK, Zhang X, Liu L, Woolums A, Crenshaw MA, Liao SF, Webby R, Epperson W, et al: Time-Dependent Proinflammatory Responses Shape Virus Interference during Coinfections of Influenza A Virus and Influenza D Virus. Viruses 2022, 14(2): 224.
- Yu J, Liu R, Zhou B, Chou TW, Ghedin E, Sheng Z, Gao R, Zhai SL, Wang D, Li F: Development and Characterization of a Reverse-Genetics System for Influenza D Virus. J Virol 2019, 93(21): e01186-19.
- Ishida H, Murakami S, Kamiki H, Matsugo H, Takenaka-Uema A, Horimoto T: Establishment of a Reverse Genetics System for Influenza D Virus. J Virol 2020, 94(10): e01767-19.
- Holwerda M, Kelly J, Laloli L, Stürmer I, Portmann J, Stalder H, Dijkman R: Determining the Replication Kinetics and Cellular Tropism of Influenza D Virus on Primary Well-Differentiated Human Airway Epithelial Cells. Viruses 2019, 11(4): 377.
- Yesilbag K, Toker EB, Ates O: Recent strains of influenza D virus create a new genetic cluster for European strains. Microb Pathog 2022, 172: 105769.
- Zhang X, Outlaw C, Olivier AK, Woolums A, Epperson W, Wan XF: Pathogenesis of co-infections of influenza D virus and Mannheimia haemolytica in cattle. Vet Microbiol 2019, 231: 246-253.
- Walz L, Kays SK, Zimmer G, von Messling V: Neuraminidase-Inhibiting Antibody Titers Correlate with Protection from Heterologous Influenza Virus Strains of the Same Neuraminidase Subtype. J Virol 2018, 92(17): e01006-18.
- Dias AS, Costa É A, Rajão DS, Guedes RM, Ciacci Zanella JR, Lobato ZI: Distribution of antibodies against influenza virus in pigs from farrow-to-finish farms in Minas Gerais state, Brazil. Influenza Other Respir Viruses 2015, 9(3): 161-167.
- Kaplan BS, Anderson TK, Chang J, Santos J, Perez D, Lewis N, Vincent AL: Evolution and Antigenic Advancement of N2 Neuraminidase of Swine Influenza A Viruses Circulating in the United States following Two Separate Introductions from Human Seasonal Viruses. J Virol 2021, 95(20): e0063221.
- Liang Y: Pathogenicity and virulence of influenza. Virulence 2023, 14(1): 2223057.
- Jiang H, Peng W, Qi J, Chai Y, Song H, Bi Y, Rijal P, Wang H, Oladejo BO, Liu J, et al: Structure-Based Modification of an Anti-neuraminidase Human Antibody Restores Protection Efficacy against the Drifted Influenza Virus. mBio 2020, 11(5): e02315-20.
- Gouma S, Anderson EM, Hensley SE: Challenges of Making Effective Influenza Vaccines. Annu Rev Virol 2020, 7(1): 495-512.
- Hayakawa J, Masuko T, Takehana T, Suzuki T: Genetic and Antigenic Characterization and Retrospective Surveillance of Bovine Influenza D Viruses Identified in Hokkaido, Japan from 2018 to 2020. Viruses 2020, 12(8): 877.
- Nguyen QT, Choi YK: Targeting Antigens for Universal Influenza Vaccine Development. Viruses 2021, 13(6): 973.
- Rajão DS, Walia RR, Campbell B, Gauger PC, Janas-Martindale A, Killian ML, Vincent AL: Reassortment between Swine H3N2 and 2009 Pandemic H1N1 in the United States Resulted in Influenza A Viruses with Diverse Genetic Constellations with Variable Virulence in Pigs. J Virol 2017, 91(4): e01763-16.
- Li C, Chen H: Enhancement of influenza virus transmission by gene reassortment. Curr Top Microbiol Immunol 2014, 385: 185-204.
- Allen JD, Jang H, DiNapoli J, Kleanthous H, Ross TM: Elicitation of Protective Antibodies against 20 Years of Future H3N2 Cocirculating Influenza Virus Variants in Ferrets Preimmune to Historical H3N2 Influenza Viruses. J Virol 2019, 93(3): e00946-18.
- Qiu Y, Stegalkina S, Zhang J, Boudanova E, Park A, Zhou Y, Prabakaran P, Pougatcheva S, Ustyugova IV, Vogel TU, et al: Mapping of a Novel H3-Specific Broadly Neutralizing Monoclonal Antibody Targeting the Hemagglutinin Globular Head Isolated from an Elite Influenza Virus-Immunized Donor Exhibiting Serological Breadth. J Virol 2020, 94(6): e01035-19.
- Sanogo IN, Kouakou C, Batawui K, Djegui F, Byarugaba DK, Adjin R, Adjabli K, Wabwire-Mangen F, Erima B, Atim G, et al: Serological Surveillance of Influenza D Virus in Ruminants and Swine in West and East Africa, 2017-2020. Viruses 2021, 13(9): 1749.
- Chen Y, Trovão NS, Wang G, Zhao W, He P, Zhou H, Mo Y, Wei Z, Ouyang K, Huang W, et al: Emergence and Evolution of Novel Reassortant Influenza A Viruses in Canines in Southern China. mBio 2018, 9(3): e00909-18.
- Saegerman C, Gaudino M, Savard C, Broes A, Ariel O, Meyer G, Ducatez MF: Influenza D virus in respiratory disease in Canadian, province of Québec, cattle: Relative importance and evidence of new reassortment between different clades. Transbound Emerg Dis 2022, 69(3): 1227-1245.
- Song Y, Zhang Y, Zhang B, Chen L, Zhang M, Wang J, Jiang Y, Yang C, Jiang T: Identification, Genetic Analysis, and Pathogenicity of Classical Swine H1N1 and Human-Swine Reassortant H1N1 Influenza Viruses from Pigs in China. Viruses 2020, 12(1): 55.
- Rajão DS, Gauger PC, Anderson TK, Lewis NS, Abente EJ, Killian ML, Perez DR, Sutton TC, Zhang J, Vincent AL: Novel Reassortant Human-Like H3N2 and H3N1 Influenza A Viruses Detected in Pigs Are Virulent and Antigenically Distinct from Swine Viruses Endemic to the United States. J Virol 2015, 89(22): 11213-11222.
- Cai M, Wei Z, Hu X, Ji Y, Li S, Huang J, Jin R, Liang Q, Zhang G, Zheng Z, et al: The evolution, complexity, and diversity of swine influenza viruses in China: A hidden public health threat. Virology 2024, 598: 110167.
- Gorin S, Richard G, Hervé S, Eveno E, Blanchard Y, Jardin A, Rose N, Simon G: Characterization of Influenza D Virus Reassortant Strain in Swine from Mixed Pig and Beef Farm, France. Emerg Infect Dis 2024, 30(8): 1672-1676.
- Liu L, Wang F, Wu Y, Mi W, Zhang Y, Chen L, Wang D, Deng G, Shi J, Chen H, et al: The V223I substitution in hemagglutinin reduces the binding affinity to human-type receptors while enhancing the thermal stability of the H3N2 canine influenza virus. Front Microbiol 2024, 15: 1442163.
- Umar S, Ma MJ, Gray GC: Influenza D virus was not detected among prospectively studied swine farm in multiple provinces of China. Influenza Other Respir Viruses 2023, 17(11): e13223.
- Glebova TI, Klivleyeva NG, Saktaganov NT, Shamenova MG, Lukmanova GV, Baimukhametova AM, Baiseiit SB, Ongarbayeva NS, Orynkhanov KA, Ametova AV, et al: Circulation of influenza viruses in the dog population in Kazakhstan (2023-2024). Open Vet J 2024, 14(8): 1896-1904.
- Mekata H, Yamamoto M, Hamabe S, Tanaka H, Omatsu T, Mizutani T, Hause BM, Okabayashi T: Molecular epidemiological survey and phylogenetic analysis of bovine influenza D virus in Japan. Transbound Emerg Dis 2018, 65(2): e355-e360.
- Anderson BD, Yondon M, Bailey ES, Duman EK, Simmons RA, Greer AG, Gray GC: Environmental bioaerosol surveillance as an early warning system for pathogen detection in North Carolina swine farms: A pilot study. Transbound Emerg Dis 2021, 68(2): 361-367.
- Sun YF, Wang XH, Li XL, Zhang L, Li HH, Lu C, Yang CL, Feng J, Han W, Ren WK, et al: Novel triple-reassortant H1N1 swine influenza viruses in pigs in Tianjin, Northern China. Vet Microbiol 2016, 183: 85-91.
- Zhao Y, Han L, Sang H, Liu S, Yang P, Hou Y, Xiao Y: Swine Influenza Viruses Isolated from 2019 to 2022 in Shandong Province, China, Exemplify the Dominant Genotype. Genes (Basel) 2024, 15(7): 849.
- Oliva J, Mettier J, Sedano L, Delverdier M, Bourgès-Abella N, Hause B, Loupias J, Pardo I, Bleuart C, Bordignon PJ, et al: Murine Model for the Study of Influenza D Virus. J Virol 2020, 94(4): e01662-19.
- Katayama M, Murakami S, Ishida H, Matsugo H, Sekine W, Ohira K, Takenaka-Uema A, Horimoto T: Antigenic commonality and divergence of hemagglutinin-esterase-fusion protein among influenza D virus lineages revealed using epitope mapping. J Virol 2024, 98(3): e0190823.
- Odagiri T, Ishida H, Li JY, Endo M, Kobayashi T, Kamiki H, Matsugo H, Takenaka-Uema A, Murakami S, Horimoto T: Antigenic heterogeneity among phylogenetic clusters of influenza D viruses. J Vet Med Sci 2018, 80(8): 1241-1244.
- Bonilla-Aldana DK, Jimenez-Diaz SD, Barboza JJ, Rodriguez-Morales AJ: Mapping the Spatiotemporal Distribution of Bovine Rabies in Colombia, 2005-2019. Trop Med Infect Dis 2022, 7(12): 406.
- Bolton JS, Klim H, Wellens J, Edmans M, Obolski U, Thompson CP: An Antigenic Thrift-Based Approach to Influenza Vaccine Design. Vaccines (Basel) 2021, 9(6): 657.
- Bonilla-Aldana DK, Jiménez-Diaz SD, Lozada-Riascos C, Silva-Cajaleon K, Rodríguez-Morales AJ: Mapping Bovine Tuberculosis in Colombia, 2001-2019. Vet Sci 2024, 11(5): 220.
- Souza CK, Anderson TK, Chang J, Venkatesh D, Lewis NS, Pekosz A, Shaw-Saliba K, Rothman RE, Chen KF, Vincent AL: Antigenic Distance between North American Swine and Human Seasonal H3N2 Influenza A Viruses as an Indication of Zoonotic Risk to Humans. J Virol 2022, 96(2): e0137421.
- Gao J, Couzens L, Burke DF, Wan H, Wilson P, Memoli MJ, Xu X, Harvey R, Wrammert J, Ahmed R, et al: Antigenic Drift of the Influenza A(H1N1)pdm09 Virus Neuraminidase Results in Reduced Effectiveness of A/California/7/2009 (H1N1pdm09)-Specific Antibodies. mBio 2019, 10(2): e00307-19.
- Chen Z, Bancej C, Lee L, Champredon D: Antigenic drift and epidemiological severity of seasonal influenza in Canada. Sci Rep 2022, 12(1): 15625.
- de Vries RD, Altenburg AF, Rimmelzwaan GF: Universal influenza vaccines, science fiction or soon reality? Expert Rev Vaccines 2015, 14(10): 1299-1301.
- Jang YH, Seong BL: The Quest for a Truly Universal Influenza Vaccine. Front Cell Infect Microbiol 2019, 9: 344.
- Nuwarda RF, Alharbi AA, Kayser V: An Overview of Influenza Viruses and Vaccines. Vaccines (Basel) 2021, 9(9): 1032.
- Kitikoon P, Knetter SM, Mogler MA, Morgan CL, Hoehn A, Puttamreddy S, Strait EL, Segers R: Quadrivalent neuraminidase RNA particle vaccine protects pigs against homologous and heterologous strains of swine influenza virus infection. Vaccine 2023, 41(47): 6941-6951.
- Zhang Z, Li S, Zhu X, Hou J, Zhang H, Zhao B, Tian Z: Increased genetic variation of A(H3N2) virus from influenza surveillance at the end of the 2016/2017 season for Shanghai port, China. Sci Rep 2022, 12(1): 17089.
- Cardenas M, Seibert B, Cowan B, Caceres CJ, Gay LC, Cargnin Faccin F, Perez DR, Baker AL, Anderson TK, Rajao DS: Modulation of human-to-swine influenza a virus adaptation by the neuraminidase low-affinity calcium-binding pocket. Commun Biol 2024, 7(1): 1230.
- Sun H, Blackmon S, Yang G, Waters K, Li T, Tangwangvivat R, Xu Y, Shyu D, Wen F, Cooley J, et al: Zoonotic Risk, Pathogenesis, and Transmission of Avian-Origin H3N2 Canine Influenza Virus. J Virol 2017, 91(21): e00637-17.
- Cui X, Ma J, Pang Z, Chi L, Mai C, Liu H, Liao M, Sun H: The evolution, pathogenicity and transmissibility of quadruple reassortant H1N2 swine influenza virus in China: A potential threat to public health. Virol Sin 2024, 39(2): 205-217.
- Peng X, Wu H, Xu L, Peng X, Cheng L, Jin C, Xie T, Lu X, Wu N: Molecular characterization of a novel reassortant H1N2 influenza virus containing genes from the 2009 pandemic human H1N1 virus in swine from eastern China. Virus Genes 2016, 52(3): 405-410.
- Makoschey B, Berge AC: Review on bovine respiratory syncytial virus and bovine parainfluenza - usual suspects in bovine respiratory disease - a narrative review. BMC Vet Res 2021, 17(1): 261.
- Crowe JE: Antibody Determinants of Influenza Immunity. J Infect Dis 2019, 219(Suppl_1): S21-s29.
- Erickson N, Waldner C, Snyder E, Araya MB, Sniatynski M, Ellis J: Comparative efficacy of modified-live and inactivated vaccines in boosting responses to bovine respiratory syncytial virus, bovine parainfluenza virus Type 3, and bovine coronavirus following neonatal mucosal priming of beef calves. Can Vet J 2024, 65(8): 791-801.
- Shi HF, Zhu YM, Dong XM, Cai H, Ma L, Wang S, Yan H, Wang XZ, Xue F: Pathogenesis of a genotype C strain of bovine parainfluenza virus type 3 infection in albino guinea pigs. Virus Res 2014, 188: 1-7.
- Sun X, Ma H, Wang X, Bao Z, Tang S, Yi C, Sun B: Broadly neutralizing antibodies to combat influenza virus infection. Antiviral Res 2024, 221: 105785.
- Sun Y, Shen Y, Zhang X, Wang Q, Liu L, Han X, Jiang B, Wang R, Sun H, Pu J, et al: A serological survey of canine H3N2, pandemic H1N1/09 and human seasonal H3N2 influenza viruses in dogs in China. Vet Microbiol 2014, 168(1): 193-196.
- Alvarez IJ, Fort M, Pasucci J, Moreno F, Gimenez H, Näslund K, Hägglund S, Zohari S, Valarcher JF: Seroprevalence of influenza D virus in bulls in Argentina. J Vet Diagn Invest 2020, 32(4): 585-588.
- Quast M, Sreenivasan C, Sexton G, Nedland H, Singrey A, Fawcett L, Miller G, Lauer D, Voss S, Pollock S, et al: Serological evidence for the presence of influenza D virus in small ruminants. Vet Microbiol 2015, 180(3-4): 281-285.
- Ducatez MF, Pelletier C, Meyer G: Influenza D virus in cattle, France, 2011-2014. Emerg Infect Dis 2015, 21(2): 368-371.
- Murakami S, Endoh M, Kobayashi T, Takenaka-Uema A, Chambers JK, Uchida K, Nishihara M, Hause B, Horimoto T: Influenza D Virus Infection in Herd of Cattle, Japan. Emerg Infect Dis 2016, 22(8): 1517-1519.
- Snoeck CJ, Oliva J, Pauly M, Losch S, Wildschutz F, Muller CP, Hübschen JM, Ducatez MF: Influenza D Virus Circulation in Cattle and Swine, Luxembourg, 2012-2016. Emerg Infect Dis 2018, 24(7): 1388-1389.
- Ferguson L, Luo K, Olivier AK, Cunningham FL, Blackmon S, Hanson-Dorr K, Sun H, Baroch J, Lutman MW, Quade B, et al: Influenza D Virus Infection in Feral Swine Populations, United States. Emerg Infect Dis 2018, 24(6): 1020-1028.
- Silveira S, Falkenberg SM, Kaplan BS, Crossley B, Ridpath JF, Bauermann FB, Fossler CP, Dargatz DA, Dassanayake RP, Vincent AL, et al: Serosurvey for Influenza D Virus Exposure in Cattle, United States, 2014-2015. Emerg Infect Dis 2019, 25(11): 2074-2080.
- Hallmann-Szelińska E, Szymański K, Łuniewska K, Kondratiuk K, Brydak LB: Hemagglutination inhibition antibody titers as a correlate of protection against influenza disease in the 2018/2019 epidemic season in Poland. Acta Biochim Pol 2020, 67(1): 93-98.
- Yu J, Wen Z, Hu W, Chen M, Zhang Y, Liu S, Wang G, Wang Z, Wang D, Zhai SL, et al: Influenza D virus infection in China, 2022-2023. Emerg Microbes Infect 2024, 13(1): 2343907.
- Murakami S, Sato R, Ishida H, Katayama M, Takenaka-Uema A, Horimoto T: Influenza D Virus of New Phylogenetic Lineage, Japan. Emerg Infect Dis 2020, 26(1): 168-171.
- Lee IW, Kim YI, Lim GJ, Kwon HI, Si YJ, Park SJ, Kim EH, Kim SM, Nguyen HD, Song MS, et al: Comparison of the virulence and transmissibility of canine H3N2 influenza viruses and characterization of their canine adaptation factors. Emerg Microbes Infect 2018, 7(1): 17.
- Haach V, Bastos APA, Gava D, da Fonseca FN, Morés MAZ, Coldebella A, Franco AC, Schaefer R: A polyvalent virosomal influenza vaccine induces broad cellular and humoral immunity in pigs. Virol J 2023, 20(1): 181.
- Wan Y, Kang G, Sreenivasan C, Daharsh L, Zhang J, Fan W, Wang D, Moriyama H, Li F, Li Q: A DNA Vaccine Expressing Consensus Hemagglutinin-Esterase Fusion Protein Protected Guinea Pigs from Infection by Two Lineages of Influenza D Virus. J Virol 2018, 92(11): e00110-18.
- Sreenivasan CC, Sheng Z, Wang D, Li F: Host Range, Biology, and Species Specificity of Seven-Segmented Influenza Viruses-A Comparative Review on Influenza C and D. Pathogens 2021, 10(12): 1583.
- Salem E, Cook EAJ, Lbacha HA, Oliva J, Awoume F, Aplogan GL, Hymann EC, Muloi D, Deem SL, Alali S, et al: Serologic Evidence for Influenza C and D Virus among Ruminants and Camelids, Africa, 1991-2015. Emerg Infect Dis 2017, 23(9): 1556-1559.
- Laloli L, Licheri MF, Probst L, Licheri M, Gultom M, Holwerda M, V'Kovski P, Dijkman R: Time-resolved characterization of the innate immune response in the respiratory epithelium of human, porcine, and bovine during influenza virus infection. Front Immunol 2022, 13: 970325.
- Feuerbaum S, Saile N, Pohlentz G, Müthing J, Schmidt H: De-O-Acetylation of mucin-derived sialic acids by recombinant NanS-p esterases of Escherichia coli O157:H7 strain EDL933. Int J Med Microbiol 2018, 308(8): 1113-1120.
- Sreenivasan CC, Liu R, Gao R, Guo Y, Hause BM, Thomas M, Naveed A, Clement T, Rausch D, Christopher-Hennings J, et al: Influenza C and D Viruses Demonstrated a Differential Respiratory Tissue Tropism in a Comparative Pathogenesis Study in Guinea Pigs. J Virol 2023, 97(6): e0035623.
- Mach N: The forecasting power of the mucin-microbiome interplay in livestock respiratory diseases. Vet Q 2024, 44(1): 1-18.
- Nogales A, Aydillo T, Ávila-Pérez G, Escalera A, Chiem K, Cadagan R, DeDiego ML, Li F, García-Sastre A, Martínez-Sobrido L: Functional Characterization and Direct Comparison of Influenza A, B, C, and D NS1 Proteins in vitro and in vivo. Front Microbiol 2019, 10: 2862.
- Morimoto R, Isegawa Y: Anti-Influenza Virus Activity of Citrullus lanatus var. citroides as a Functional Food: A Review. Foods 2023, 12(20): 3866.
- Lanave G, Camero M, Coppola C, Marchi S, Cascone G, Salina F, Coltraro M, Odigie AE, Montomoli E, Chiapponi C, et al: Serological Evidence for Circulation of Influenza D Virus in the Ovine Population in Italy. Pathogens 2024, 13(2): 162.
- Trombetta CM, Montomoli E, Di Bartolo I, Ostanello F, Chiapponi C, Marchi S: Detection of antibodies against influenza D virus in swine veterinarians in Italy in 2004. J Med Virol 2022, 94(6): 2855-2859.
- Wan XF, Ferguson L, Oliva J, Rubrum A, Eckard L, Zhang X, Woolums AR, Lion A, Meyer G, Murakami S, et al: Limited Cross-Protection Provided by Prior Infection Contributes to High Prevalence of Influenza D Viruses in Cattle. J Virol 2020, 94(18): e00240-20.
- Ferguson L, Eckard L, Epperson WB, Long LP, Smith D, Huston C, Genova S, Webby R, Wan XF: Influenza D virus infection in Mississippi beef cattle. Virology 2015, 486: 28-34.
- Goecke NB, Liang Y, Otten ND, Hjulsager CK, Larsen LE: Characterization of Influenza D Virus in Danish Calves. Viruses 2022, 14(2): 423.
- Masset N, Meurens F, Marie M, Lesage P, Lehébel A, Brisseau N, Assié S: Effectiveness of two intranasal vaccines for the control of bovine respiratory disease in newborn beef calves: A randomized non-inferiority multicentre field trial. Vet J 2020, 263: 105532.
- Fekete E, Buret AG: The role of mucin O-glycans in microbiota dysbiosis, intestinal homeostasis, and host-pathogen interactions. Am J Physiol Gastrointest Liver Physiol 2023, 324(6): G452-g465.
- Foni E, Chiapponi C, Baioni L, Zanni I, Merenda M, Rosignoli C, Kyriakis CS, Luini MV, Mandola ML, Bolzoni L, et al: Influenza D in Italy: towards a better understanding of an emerging viral infection in swine. Sci Rep 2017, 7(1): 11660.
- Luo J, Ferguson L, Smith DR, Woolums AR, Epperson WB, Wan XF: Serological evidence for high prevalence of Influenza D Viruses in Cattle, Nebraska, United States, 2003-2004. Virology 2017, 501: 88-91.
- Chiapponi C, Faccini S, Fusaro A, Moreno A, Prosperi A, Merenda M, Baioni L, Gabbi V, Rosignoli C, Alborali GL, et al: Detection of a New Genetic Cluster of Influenza D Virus in Italian Cattle. Viruses 2019, 11(12): 1110.
- Gorin S, Fablet C, Quéguiner S, Barbier N, Paboeuf F, Hervé S, Rose N, Simon G: Assessment of Influenza D Virus in Domestic Pigs and Wild Boars in France: Apparent Limited Spread within Swine Populations Despite Serological Evidence of Breeding Sow Exposure. Viruses 2019, 12(1): 25.
- Kowalczyk D, Szymański K, Cieślak K, Hallmann-Szelińska E, Brydak LB: Circulation of Influenza Virus in the 2015/2016 Epidemic Season in Poland: Serological Evaluation of Anti-hemagglutinin Antibodies. Adv Exp Med Biol 2019, 1150: 77-82.
- Junqueira DM, Tochetto C, Anderson TK, Gava D, Haach V, Cantão ME, Baker ALV, Schaefer R: Human-to-swine introductions and onward transmission of 2009 H1N1 pandemic influenza viruses in Brazil. Front Microbiol 2023, 14: 1243567.
- Yu J, Li T, Wen Z, Wu S, Wang Z, Zheng J, Chen M, Chen F, Wei WK, Zhai SL, et al: Identification of D/Yama2019 Lineage-Like Influenza D Virus in Chinese Cattle. Front Vet Sci 2022, 9: 939456.
- Uprety T, Yu J, Nogales A, Naveed A, Yu H, Chen X, Liu Y, Bowman AS, Martinez-Sobrido L, Parrish CR, et al: Influenza D virus utilizes both 9-O-acetylated N-acetylneuraminic and 9-O-acetylated N-glycolylneuraminic acids as functional entry receptors. J Virol 2024, 98(3): e0004224.
- Liu R, Sreenivasan C, Yu H, Sheng Z, Newkirk SJ, An W, Smith DF, Chen X, Wang D, Li F: Influenza D virus diverges from its related influenza C virus in the recognition of 9-O-acetylated N-acetyl- or N-glycolyl-neuraminic acid-containing glycan receptors. Virology 2020, 545: 16-23.
- Sreenivasan C, Thomas M, Sheng Z, Hause BM, Collin EA, Knudsen DE, Pillatzki A, Nelson E, Wang D, Kaushik RS, et al: Replication and Transmission of the Novel Bovine Influenza D Virus in a Guinea Pig Model. J Virol 2015, 89(23): 11990-12001.
- Gaudino M, Chiapponi C, Moreno A, Zohari S, O'Donovan T, Quinless E, Sausy A, Oliva J, Salem E, Fusade-Boyer M, et al: Evolutionary and temporal dynamics of emerging influenza D virus in Europe (2009-22). Virus Evol 2022, 8(2): veac081.
- Lim EH, Lim SI, Kim MJ, Kwon M, Kim MJ, Lee KB, Choe S, An DJ, Hyun BH, Park JY, et al: First Detection of Influenza D Virus Infection in Cattle and Pigs in the Republic of Korea. Microorganisms 2023, 11(7): 1751.
- Kwasnik M, Rola J, Rozek W: Influenza D in Domestic and Wild Animals. Viruses 2023, 15(12): 2433.
- Nedland H, Wollman J, Sreenivasan C, Quast M, Singrey A, Fawcett L, Christopher-Hennings J, Nelson E, Kaushik RS, Wang D, et al: Serological evidence for the co-circulation of two lineages of influenza D viruses in equine populations of the Midwest United States. Zoonoses Public Health 2018, 65(1): e148-e154.
- Gaudino M, Moreno A, Snoeck CJ, Zohari S, Saegerman C, O'Donovan T, Ryan E, Zanni I, Foni E, Sausy A, et al: Emerging Influenza D virus infection in European livestock as determined in serology studies: Are we underestimating its spread over the continent? Transbound Emerg Dis 2021, 68(3): 1125-1135.
- Salem E, Hägglund S, Cassard H, Corre T, Näslund K, Foret C, Gauthier D, Pinard A, Delverdier M, Zohari S, et al: Pathogenesis, Host Innate Immune Response, and Aerosol Transmission of Influenza D Virus in Cattle. J Virol 2019, 93(7): e01853-18.
Asia-Pacific Journal of Pharmacotherapy & Toxicology
p-ISSN: 2788-6840
e-ISSN: 2788-6859
Copyright © Asia Pac J Pharmacother Toxicol. This work is licensed under a Creative Commons Attribution-NonCommercial-No Derivatives 4.0 International (CC BY-NC-ND 4.0) License.