Review Article | Open Access
Respiratory syncytial virus (RSV): prevention and antiviral therapeutic strategies
Shahnaz Parveen1, Shaikh Salam1
1School and Building of Pharmaceutical Sciences, Plot 3, North South University, Bashundhara District, NSU road, Dhaka-1229, Bangladesh.
Correspondence: Shaikh Salam (School and Building of Pharmaceutical Sciences, Plot 3, North South University, Bashundhara District, NSU road, Dhaka-1229, Bangladesh; E-mail: shaikh.salam@northsouth.edu).
Asia-Pacific Journal of Pharmacotherapy & Toxicology 2025, 5: 22-32. https://doi.org/10.32948/ajpt.2025.02.08
Received: 06 Dec 2024 | Accepted: 19 Feb 2025 | Published online: 21 Feb 2025
Key words antivirals, RSV, immune responses, prevention, treatment, vaccine
The immune system primarily focuses on RSV's surface glycoproteins—most notably the G and F proteins [6]. To distinguish between RSV groups, serum-neutralizing antibodies, along with mucosal IgA and IgG, target the glycoprotein G, and this recognition varies depending on the strain [7]. On the other hand, RSV groups A and B exhibit cross-reactivity in antibodies against glycoprotein F. IgA is essential for protecting the upper respiratory tract because it supports both long-term immunity during reinfection and the immediate response after a first infection [8]. In the early stages of an infant's initial RSV infection, the IgG antibodies inherited from the mother can obstruct the formation of a localized IgA response in the respiratory tract [9]. While circulating IgG does offer a degree of defense against RSV, its brief duration in the bloodstream, combined with the virus's effective immune evasion tactics, frequently leads to repeated infections [10]. In this review, we examine the current clinically available therapies and prophylactic agents for RSV. We specifically focus on treatments that are tailored for RSV, as well as non-specific agents and approaches that have been explored for RSV management. Additionally, we discuss various strategies aimed at preventing RSV infection.
In 1987, researchers established that the G protein plays a crucial role as an attachment molecule [16]. About 60% of its structure is composed of carbs, and it is highly glycosylated. There are two forms of this protein: one that is released and one that is membrane-bound, which helps RSV attach to respiratory epithelial cells [17]. The RSV structural element that varies the most is its full-length transmembrane form, which is classified as a type II integral membrane protein. Despite its variability, it contains a conserved central domain of 26 amino acids that plays a critical role in RSV pathophysiology and notably lacks glycosylation. In contrast, while the G protein is less effective in eliciting neutralizing antibodies, the F protein stands out as a highly conserved type I integral membrane glycoprotein that is essential for mediating viral entry. It facilitates membrane fusion between nearby infected cells and the virus as well as between the virus and host cells [18]. The protein exists in two distinct shapes: one is a stable pre-fusion form, and the other is a very stable post-fusion form that appears once the virus attaches to the host cell [19]. The F protein carries six key antigenic sites, designated as Ø and I–V (see Table 1). Specifically, epitopes I, II, and IV are present in both the pre- and post-fusion states, whereas epitopes Ø, III, and V are only exposed in the pre-fusion form [20].
Table 1. Characteristics of RSV F fragments. |
|||
RSV F configuration |
Position name |
Structural and functional features |
Product |
pre-F |
θ
|
Contains an α-helical segment from the F1 region (amino acids 196–210) combined with a β-strand from the F2 region (amino acids 62–69). This site is targeted by antibodies such as D25 and AM22. |
Nirsevimab, RSM01 (currently in clinical development) |
post-F
|
I
|
Located within the F2 subunit, near residue Pro389, also identified as the 131-2a region. |
- |
pre-F and post-F |
II |
Features a helix-turn-helix motif from the F1 region (amino acids 253–278) that includes a conserved epitope recognized by a mota-specific antibody. |
Previously targeted by Palivizumab and Motavizumab (now discontinued) |
pre-F and post-F |
III |
Comprises two anti-parallel β-strands and is recognized by the MPE8-specific antibody. |
- |
pre-F and post-F |
IV |
This segment contains a linear epitope (amino acids 422–436) recognized by 101F-targeted antibodies, which, however, do not interfere with viral attachment. |
Clesrovimab (currently in clinical development) |
pre-F |
V |
Encompassing regions α2–α3 and β3–β4, this portion lies between antigenic sites Ø and III on the pre-fusion RSV F protein and is recognized by AM14-specific antibodies. |
Suptavumab (development discontinued) |
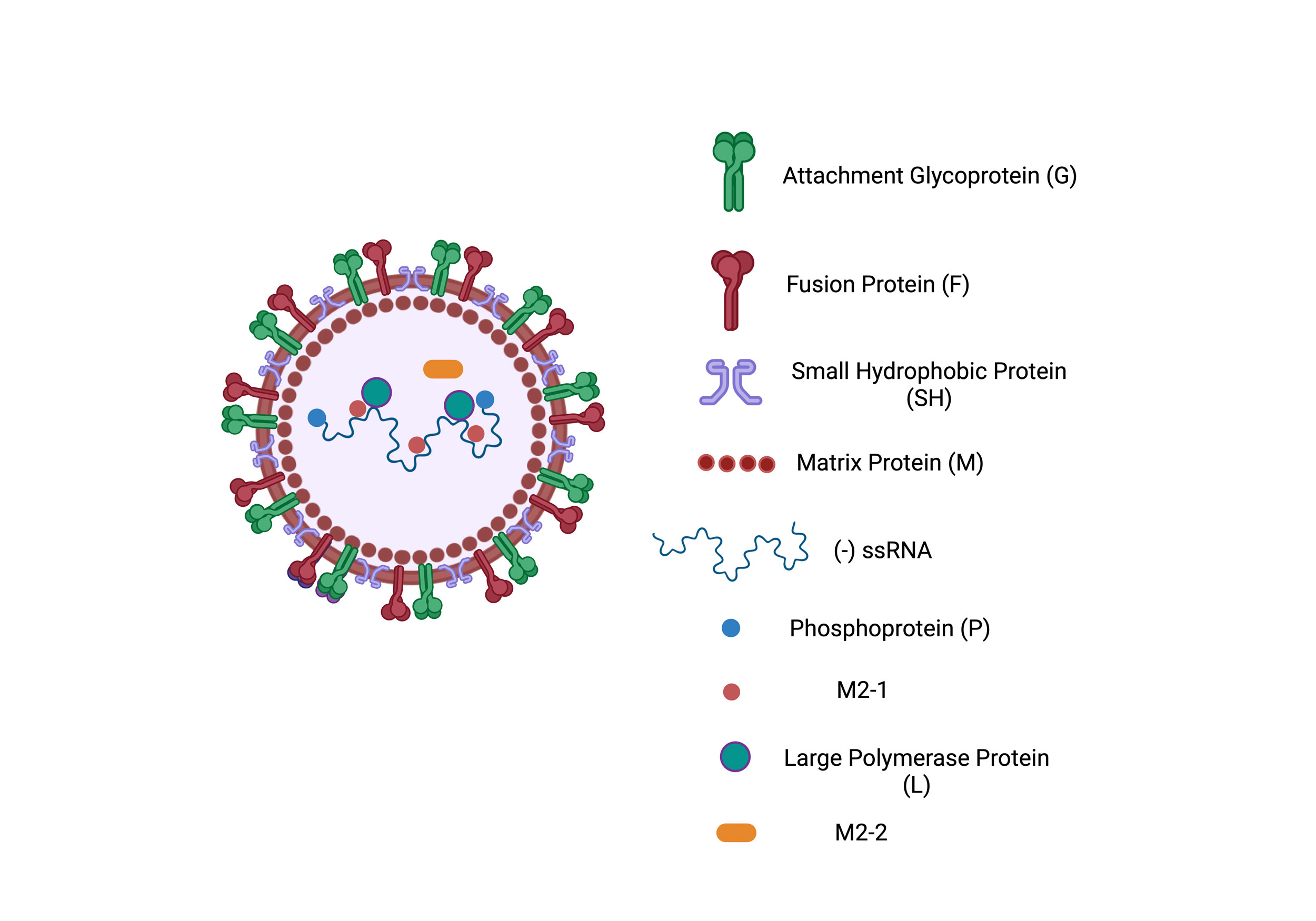
By blocking important functional proteins involved in each stage of the viral replication cycle, antiviral therapies target different stages of the cycle. Some of these treatments target proteins that help the virus connect to receptors on the host cell, blocking fusion and cell entry and others target non-structural proteins of viruses that are essential for replication [25]. To lower the possibility of medication resistance, host-directed therapies, in addition to direct-acting antivirals, work to block cellular elements necessary for the viral life cycle. In order to more successfully remove viruses, these therapies function by inhibiting particular enzymes, stopping viral entry and assembly, and strengthening the host's immune system [26].
Targeting viruses with a protein envelope, viral protease inhibitors are another crucial family of antivirals. These inhibitors work by interfering with the protease enzymes that process viral polyproteins, a critical step for the virus to form both its structural and non-structural proteins. By doing so, they disrupt the assembly of the virus. Moreover, by preventing the production of key structural proteins, they effectively block the formation of new viral particles, thereby halting viral replication [27]. Because they obstruct the enzymes that cause viral DNA or RNA replication, inhibitors of genetic material replication are also essential. By blocking the virus's ability to duplicate its genetic material inside host cells, this method proves effective against both DNA and RNA viruses, showcasing the diverse strategies in antiviral drug development [28] (Figure 3).
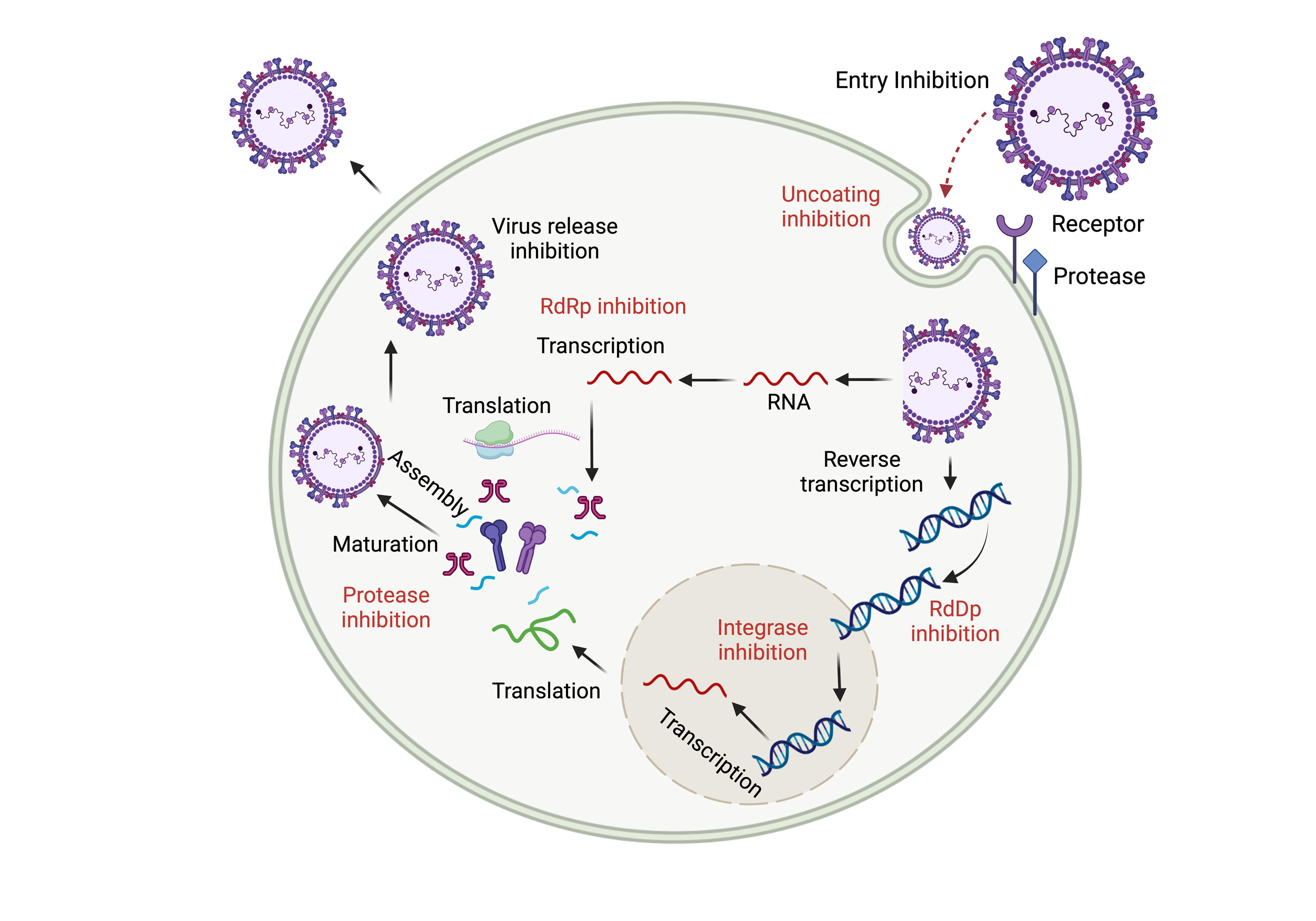
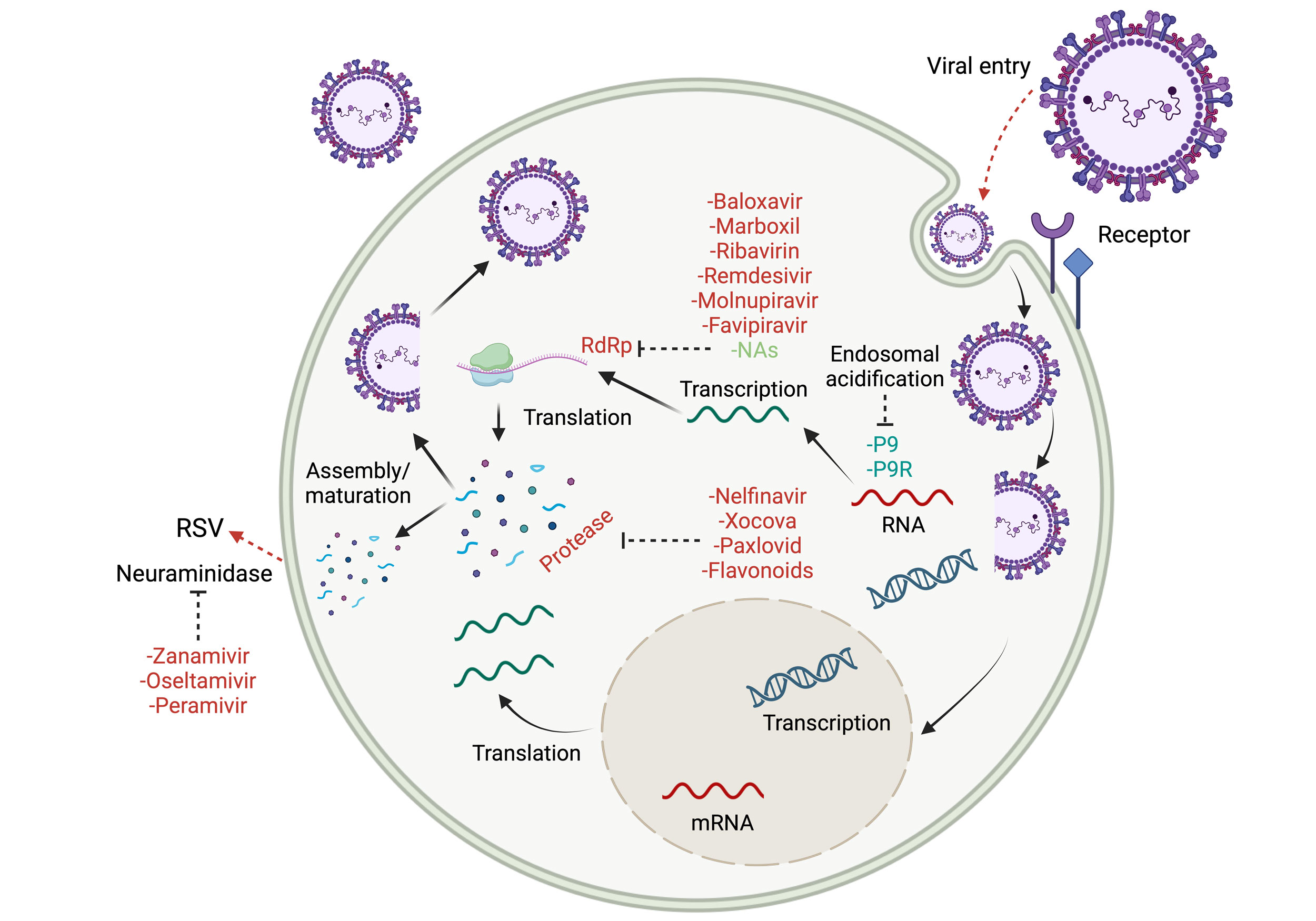
Ribavirin, a nucleoside analog with broad-spectrum activity, works by preventing the replication of both DNA and RNA viruses. It can be administered in various ways, such as orally, through IV injection, or via aerosol. However, an earlier Cochrane review—which analyzed 12 randomized trials comparing ribavirin to a placebo in infants and young children with RSV-related lower respiratory tract infections—did not find a significant reduction in mortality (OR 0.37; 95% CI, 0.12 to 1.18). No significant differences were observed between the treatment and placebo groups regarding secondary endpoints, disease severity, or oxygenation improvements [29]. The aerosolized form of ribavirin relies on specialized inhalation devices, notably the SPAG-2 small-particle aerosol generator (model-2) [30].
A randomized controlled trial was conducted with bone marrow transplant patients who had tested positive for RSV [31]. Participants who met the eligibility requirements were randomized to receive supportive care alone or aerosolized ribavirin in combination. For ten days, the patient received two grams of ribavirin inhalation solution, which had a 60 mg/mL concentration, three times a day for two hours. According to the study, ribavirin treatment was linked to a decreased risk of pneumonia and viral load. Skin rash, conjunctivitis, chest pain, shortness of breath, and bronchospasm were among the side effects that were reported. Despite using the right delivery techniques, several family members and healthcare professionals reported having additional adverse effects as headaches and vomiting. Urinary concentrations were collected following shifts to evaluate healthcare workers' airborne exposure to ribavirin. In 12% of respiratory therapists' and 62% of nurses' samples, ribavirin was found. Research on animals, notably rats and rabbits, has shown that a ribavirin dose of 1 mg/kg may cause birth defects. In contrast, lower doses—around 0.3 mg/kg (which roughly translates to 0.05 mg/kg when adjusted for an adult's body surface area)—do not appear to have these harmful effects. Despite the absence of teratogenic outcomes in the children of healthcare workers exposed to aerosolized ribavirin, concerns about its potential risks remain [32].
A previous investigation evaluated the application of oral ribavirin in lung transplant recipients suffering from RSV infection [33]. In this study, 52 patients initially received a loading dose of intravenous ribavirin at 33 mg/kg divided into three separate administrations, while two others were given an equivalent dosage orally. Subsequently, 21 patients continued treatment with oral ribavirin at a dosage of 20 mg/kg administered in two doses daily, with the treatment duration ranging from 6 to 31 days (a median of 11 days). The results indicate that using oral ribavirin could serve as a practical alternative to the intravenous form for treating RSV in lung transplant recipients. Furthermore, ribavirin treatment significantly decreased mortality among patients with hematological disorders, according to a meta-analysis and comprehensive review [34]. Administering ribavirin by mouth has been demonstrated to be a safe, cost-effective, and user-friendly treatment option. Furthermore, it has proven effective in eliminating the virus.
Nevertheless, because of its overprice and possible adverse effects, ribavirin is not advised for children who are otherwise healthy. It has showed promise in critically immunocompromised populations, which is where its clinical usage is still being studied. Other antiviral drugs that target fusion or replication inhibition as their mechanisms of action, such as RV521 [35] and AK0529 [36], are also being studied in ongoing randomized controlled studies.
Palivizumab
In 1998, a breakthrough in RSV prevention was achieved with the approval of palivizumab—a recombinant, humanized monoclonal antibody designed specifically for high-risk patients. This therapeutic agent works by binding to the virus’s fusion protein, thereby blocking its ability to infect cells. Researchers have looked into its possible application in treating acute RSV infections because of its demonstrated effectiveness in prevention. Palivizumab (15 mg/kg) or a placebo was given to 420 infants with RSV bronchiolitis who had previously been healthy as part of a double-blinded randomized controlled study (RCT) [37]. The study revealed that the frequency of hospital readmissions and the length of hospital stays were nearly identical for patients treated with palivizumab compared to those given a placebo. Similarly, no statistically significant differences in clinical outcomes were observed between the two groups in another multicenter RCT that randomly allocated palivizumab or placebo to babies with acute bronchiolitis who tested positive for RSV [38].
Motavizumab
Motavizumab, a second-generation, humanized monoclonal antibody derived from palivizumab, has been investigated for its potential to treat RSV. In one multicenter randomized controlled trial, pediatric patients with RSV were randomly assigned to receive either 30 mg/kg or 100 mg/kg of motavizumab, or a placebo [39]. Over a follow-up period of 12 months, the trial found no significant differences between the groups in terms of hospitalization duration, illness severity, or the rate of subsequent wheezing episodes.
Research has explored the use of RSV-IVIG as an alternative therapeutic approach for managing acute RSV infections. Intravenous polyclonal immunoglobulin, which is made by separating RSV antibodies from pooled blood, has demonstrated potent anti-RSV properties in vivo. In addition, RSV-IVIG effectively curbs viral multiplication in lung tissue in vivo. This reduction in viral activity translates to a decreased overall viral burden, which may, in turn, prevent the emergence of respiratory illnesses [40]. In a double-blinded randomized controlled trial, 107 high-risk children under two years old, suffering from conditions such as prematurity, congenital heart disease, or chronic lung disorders, were evaluated for their response to RSV-IGIV treatment for RSV infection. One group received a single intravenous infusion of RSV-IGIV at a dosage of 1500 mg/kg, while the comparison group was administered a matching placebo. The study ultimately found that the duration of hospital stays and the overall severity of the illness did not differ significantly between the two groups [38]. Concerns regarding probable blood-borne product transfer and potential interference with routine live vaccination delivery are raised by the massive intravenous dose of RSV-IGIV. A 2019 Cochrane review that analyzed seven studies involving 486 infants with RSV bronchiolitis concluded that there wasn’t sufficient evidence to show that immunoglobulin treatment led to different outcomes compared to a placebo [41]. Later, as more effective alternatives emerged, RSV-IGIV was voluntarily withdrawn from the market in 2003 [42].
Non-specific RSV treatments
It is essential to comprehend the biology of acute infection when researching non-specific treatments for RSV bronchiolitis or infection. RSV begins its journey by infecting and multiplying along the mucosal surfaces of the respiratory tract, from the nasopharynx all the way to the smallest alveoli. In adults and older children, this often results in symptoms typical of the common cold, such as a runny nose and nasal congestion. However, the illness primarily affects the lower respiratory tract in younger children, especially newborns and preschool-aged children, which can result in more serious and sometimes fatal consequences including bronchiolitis. This variation in intensity is thought to be caused by a number of causes. One important consideration is the size of the airways: an adult's respiratory bronchiole has an average diameter of about 250 μm, but a 4-month-old infant's only has 120 μm [43]. During viral infections, this much smaller bronchiolar lumen is more prone to blockage [44]. In newborns, the risk of obstruction is heightened because their collateral ventilation pathways, specifically the pores of Kohn and the canals of Lambert, have not yet reached full maturity, unlike those found in older children and adults [45]. Due to these structural variations and their developing immune systems, newborns are more susceptible to bronchiolitis episodes that are clinically significant.
Studies conducted on lung tissue from infants who succumbed to severe RSV infections have shed light on the underlying processes of RSV bronchiolitis. Histological studies reveal that the most significant changes occur within the medium and small bronchioles (those with diameters of 150 μm or less). These alterations include airway swelling, damage to the epithelial cells accompanied by a buildup of inflammatory cells and cellular debris, as well as an increase in mucus production—all of which contribute to the obstruction of the airways [46]. Higher airway resistance brought on by this obstruction causes hyperinflation, hypoxemia, crackles, wheezing, and an increase in respiratory effort.
Normally, mucus lines the airways as a protective substance that traps particulates that are inhaled. Cilia, enveloped in periciliary fluid, beat in unison to drive mucus upward toward the upper respiratory tract. This coordinated action, coupled with an effective cough, forms the body's primary defense against airborne pathogens and particulate matter. This mechanism is compromised in bronchiolitis, in part because of the overproduction of mucus and in part because of viral damage to the cilia. Multiple studies have identified genes connected to inflammatory pathways that appear to play a role in the severe manifestations of RSV infection in infants [47]. Furthermore, the complex interaction between the virus's genetic material and the host's immune response may contribute to an increase in airway obstruction. [48]. Understanding these processes has influenced the creation of several treatment strategies meant to reverse airway blockage, such as bronchodilators, anti-inflammatory drugs and mucolytics [49], which are included in Table 2. Even with these molecular connections, the evidence does not always support the effectiveness of these drugs in treating RSV infections.
Dornase alfa
In cases of RSV bronchiolitis, mucus plugs are found to contain high concentrations of extracellular DNA, a substance released during the influx and subsequent disintegration of leukocytes [50]. This extracellular DNA is broken down by the enzyme human recombinant DNase (hrDNase), which lowers mucus viscosity. Because of this activity, hrDNase has been proposed as a possible bronchiolitis therapy. In the most extensive randomized, double-blind controlled trial to date, encompassing 225 infants hospitalized with bronchiolitis, researchers observed no notable reduction in either the duration of hospitalization or the period requiring supplemental oxygen. This outcome contrasts with earlier, smaller-scale studies and case reports that suggested improvements in radiographic signs of atelectasis among severely affected infants [51, 52]. A 2012 Cochrane review examined three studies, including the one where infants with viral bronchiolitis received hrDNase, involving a total of 333 children under 24 months. The review concluded that the treatment did not yield any significant benefits [53]. Therefore, although nebulized hrDNase is not usually advised for the treatment of RSV infection, it may be taken into consideration in cases of severe atelectasis linked to bronchiolitis when traditional treatments have failed.
N-acetylcysteine (NAC)
A substance called NAC has antioxidant qualities and dissolves the disulfide bonds in mucins, both of which may help treat bronchiolitis [54]. In an in vitro model using alveolar type-II epithelial cells infected with RSV, NAC significantly reduced both mucin production and the synthesis of pro-inflammatory mediators [55]. A randomized controlled study compared nebulized NAC with nebulized salbutamol in 100 infants. However, the trial, which may have been underpowered, failed to show significant differences in hospitalization duration or symptom severity [56]. Consequently, further research is needed before NAC can be considered a promising treatment option for RSV infection.
Nebulized hypertonic saline
Research indicates that administering nebulized hypertonic saline can significantly enhance mucociliary clearance in both healthy individuals and patients with respiratory conditions such as cystic fibrosis, bronchiectasis, and asthma. It may also lessen airway edema by generating an osmotic gradient that pulls water from the mucosa and submucosa into the mucus layer [57]. When paired with a productive cough, this hydration of the mucus layer aids in secretion mobilization and is believed to reduce airway blockage [58]. Despite these theoretical explanations, clinical evaluations and computed acoustic airflow techniques have not demonstrated that using 3% hypertonic saline improves wheeze or airflow [59].
Multiple clinical studies examining hypertonic saline as a treatment for viral bronchiolitis have not produced the anticipated positive outcomes. Although some research suggested that 3% hypertonic saline had a slight impact on hospital stay duration and symptom scores [60], a Cochrane meta-analysis in 2017, reviewing 28 trials involving 4,195 infants with acute bronchiolitis, concluded that the available evidence does not sufficiently support its use. The length of stay was somewhat shortened, nevertheless, and there were very few mild adverse events, according to the authors [60]. Subsequent randomized trials failed to show any improvement when 3% hypertonic saline was used instead of the standard supportive care [61]. Therefore, hypertonic saline is not part of the current standard of therapy for RSV bronchiolitis.
Bronchodilators
Infants with bronchiolitis often present with symptoms such as wheezing, a prolonged phase of exhalation, and distinct crackling sounds during chest examinations. Medications like salbutamol and albuterol, which are β-2 adrenergic agonists, work by relaxing the airway's smooth muscles to relieve constriction [62]. While initial meta-analyses suggested that these drugs might offer moderate short-term benefits in mild to moderate cases, a 2014 Cochrane review of 30 studies involving 1,992 infants found no significant improvements in oxygen saturation, hospitalization frequency, or length of hospital stay. Moreover, the use of these agents is associated with side effects including rapid heart rate, decreased oxygen levels, tremors, and electrolyte disturbances, leading to the general recommendation against their routine use in treating bronchiolitis, however they can be tested on infants whose primary symptom is wheezing in order to gauge the response. Notwithstanding the paucity of information, the many manifestations and symptoms of RSV infection are widely known, and future randomized clinical trials should benefit from focusing bronchodilator use on the clinical phenotype [63].
Epinephrine
Epinephrine exerts its effect by binding to β-2 adrenergic receptors, which in turn triggers the relaxation of the smooth muscles lining the air passages. Hence, it may be useful in treating acute bronchiolitis. Furthermore, its potent α-adrenergic actions result in decreased airway edema and vasoconstriction. According to a short trial, racemic epinephrine improved clinical ratings and decreased airway resistance in infants with bronchiolitis more effectively than salbutamol [64]. Nine studies (including 1354 infants) comparing nebulized epinephrine to a placebo were included in a 2011 Cochrane Review, which found that outpatients experienced a little short-term improvement. By day 7, however, the treatment had no effect on the illness's course, and neither shorter hospital stays nor better symptom scores for inpatients were the outcomes [65]. Crucially, the studies showed few side effects and acceptable tolerance. Therefore, epinephrine is not advised as a regular treatment for RSV infections, even though it can be administered in the acute phase.
Glucocorticoids
According to a number of studies, glucocorticoids have little anti-inflammatory effect when RSV infection is present, especially when it comes to viral load and cytokine production [66]. Clinical trials have not demonstrated a meaningful impact of glucocorticoids on improving clinical scores, reducing hospitalization rates, or shortening hospital stays. This ineffectiveness may be attributed to the dominant neutrophilic inflammation in RSV infections, which is known to respond poorly to glucocorticoid treatment [67, 68]. Additionally, a six-year follow-up study conducted in the Netherlands involving 185 infants found no evidence to support the claim that inhaled glucocorticoids decrease the likelihood of developing asthma later in life among newborns affected by RSV [69]. Steroids may be helpful for infants with RSV in certain situations, though, such as those who have underlying medical disorders like asthma or bronchopulmonary dysplasia. In comparison to other children infected with RSV, these groups might exhibit a greater level of steroid-responsive inflammation.
Leukotriene receptor antagonists
Leukotrienes, which interact with bronchial smooth muscle to induce bronchoconstriction, are thought to play a role in the bronchial hyperresponsiveness and mucus hypersecretion brought on by RSV [70]. A Cochrane review evaluated the impact of leukotriene receptor antagonists using data from five randomized, placebo-controlled studies involving 1,296 hospitalized infants under the age of two with bronchiolitis. The analysis determined that the current high-quality evidence is insufficient to definitively conclude whether these medications affect clinical severity scores or the duration of hospital stays [71]. It is not possible to prescribe this class of drugs for the treatment of RSV infections until more research is done.
Table 2. Methods for treating non-specific acute RSV bronchiolitis. |
|||
Product |
Mechanism of action |
Feedback |
Reference |
Bronchodilators
|
|
|
|
Salbutamol, albuterol, etc. |
Nebulized solution; β-2 adrenergic receptor agonist, which opens airways and relaxes smooth muscle. |
Not recommended. |
[49] |
Epinephrine |
Nebulized solution; A few consequences of β-2 adrenergic airway edema is also reduced by vasoconstriction brought on the α-1 adrenergic receptor actions. |
Not recommended; May reduce risk of hospitalization in the ED setting. |
[75, 66] |
Mucus therapies |
|
|
|
3% hypertonic saline |
Nebulized solution; Enhances ciliary action by generating an osmotic gradient and drawing water into the mucus layer, cough stimulation. |
Not recommended for inpatient management; May reduce the risk of hospitalization in the ED setting. |
[41] |
N-acetylcysteine |
Nebulized solution; Mucolytic substance, hydrolyzes mucus protein disulfide bonds. |
Insufficient data - not recommended. |
[76] |
Deoxyribonuclease (hrDNase) |
Mucolytic substance with nebulized solution; Breaks down extracellular DNA. |
Not recommended; In severe circumstances, when traditional therapy fails, it could be taken into consideration as a treatment option for atelectasis. |
[54, 51] |
Therapies targeting inflammation |
|
|
|
Leukotriene inhibitors |
Oral solution |
Poor evidence, not recommended. |
[71] |
Glucocorticoids |
Oral solution, inhaled or nebulized preparations; Anti-inflammatory with a broad spectrum, inhibit the production of cytokines that promote inflammation. |
Not recommended; When reactive airway disease is highly suspected, it might be taken into consideration. |
[69, 68] |
Manual therapies |
|
|
|
Chest physiotherapy |
Chest percussion, suction; Facilitates the removal of secretions, believed to lessen the ventilatory effort required for babies with the most severe illnesses. |
Not routinely recommended; When pertinent comorbidities exist, it may be taken into consideration. |
[77, 74] |
No applicable.
Ethics approval
No applicable.
Data availability
The data will be available upon request.
Funding
None.
Authors’ contribution
Shahnaz Parveen contributed to draft, critical revision of the article, table making, and figure production; Shaikh Salam revised the manuscript and approved the final submission.
Competing interests
The authors declare no competing interests.
- Shi T, McAllister DA, O'Brien KL, Simoes EA, Madhi SA, Gessner BD, Polack FP, Balsells E, Acacio S, Aguayo C et al: Global, regional, and national disease burden estimates of acute lower respiratory infections due to respiratory syncytial virus in young children in 2015: a systematic review and modelling study. Lancet 2017, 390(10098): 946-958.
- Glezen WP, Taber LH, Frank AL, Kasel JA: Risk of primary infection and reinfection with respiratory syncytial virus. Am J Dis Child 1986, 140(6): 543-546.
- Morris JA, Blount Jr RE, Savage R: Recovery of cytopathogenic agent from chimpanzees with goryza. Proc Soc Exp Biol Med 1956, 92(3): 544-549.
- Adams JM, Imagawa DT, Zike K: Epidemic bronchiolitis and pneumonitis related to respiratory syncytial virus. JAMA 1961, 176(12): 1037-1039.
- Afonso CL, Amarasinghe GK, Bányai K, Bào Y, Basler CF, Bavari S, Bejerman N, Blasdell KR, Briand FX, Briese T et al: Taxonomy of the order Mononegavirales: update 2016. Arch Virol 2016, 161(8): 2351-2360.
- McNamara PS, Fonceca AM, Howarth D, Correia JB, Slupsky JR, Trinick RE, Al Turaiki W, Smyth RL, Flanagan BF: Respiratory syncytial virus infection of airway epithelial cells, in vivo and in vitro, supports pulmonary antibody responses by inducing expression of the B cell differentiation factor BAFF. Thorax 2013, 68(1): 76-81.
- Sande CJ, Mutunga MN, Medley GF, Cane PA, Nokes DJ: Group-and genotype-specific neutralizing antibody responses against respiratory syncytial virus in infants and young children with severe pneumonia. J Infect Dis 2013, 207(3): 489-492.
- Freiborst J, Ogra PL: Mucosal immunity and viral infections. Ann Med 2001, 33(3): 172-177.
- Crowe JE, Firestone CY, Murphy BR: Passively acquired antibodies suppress humoral but not cell-mediated immunity in mice immunized with live attenuated respiratory syncytial virus vaccines. J Immunol 2001, 167(7): 3910-3918.
- Attaianese F, Guiducci S, Trapani S, Barbati F, Lodi L, Indolfi G, Azzari C, Ricci S: Reshaping Our Knowledge: Advancements in Understanding the Immune Response to Human Respiratory Syncytial Virus. Pathogens 2023, 12(9): 1118.
- Morris JA, Blount Jr RE, Savage R: Recovery of cytopathogenic agent from chimpanzees with goryza. Proc Soc Exp Biol Med 1956, 92(3): 544-549.
- Chanock R, Roizman B, Myers R: Recovery from infants with respiratory illness of a virus related to chimpanzee coryza agent (CCA) Isolation, properties and characterization. American J Epidemiol 1957, 66(3): 281-290.
- Afonso CL, Amarasinghe GK, Bányai K, Bào Y, Basler CF, Bavari S, Bejerman N, Blasdell KR, Briand FX, Briese T et al: Taxonomy of the order Mononegavirales: update 2016. Arch Virol 2016, 161(8): 2351-2360.
- Lee WanJi LW, Kim YouJin KY, Kim DaeWon KD, Lee HanSaem LH, Lee HoYeon LH, Kim KiSoon KK: Complete genome sequence of human respiratory syncytial virus genotype A with a 72-nucleotide duplication in the attachment protein G gene. J Virol 2012 86(24): 13810-13811.
- Collins PL, Fearns R, Graham BS: Respiratory syncytial virus: virology, reverse genetics, and pathogenesis of disease. Curr Top Microbiol Immunol 2013, 372: 3-38.
- Levine S, Klaiber-Franco R, Paradiso PR: Demonstration that glycoprotein G is the attachment protein of respiratory syncytial virus. J Gen Virol 1987, 68(9): 2521-2524.
- Bukreyev A, Yang L, Fricke J, Cheng L, Ward JM, Murphy BR, Collins PL: The secreted form of respiratory syncytial virus G glycoprotein helps the virus evade antibody-mediated restriction of replication by acting as an antigen decoy and through effects on Fc receptor-bearing leukocytes. J Virol 2008, 82(24): 12191-12204.
- McLellan JS, Ray WC, Peeples ME: Structure and function of respiratory syncytial virus surface glycoproteins. Challenges and opportunities for respiratory syncytial virus vaccines. Curr Top Microbiol Immunol 2013, 372: 83-104.
- Swanson KA, Settembre EC, Shaw CA, Dey AK, Rappuoli R, Mandl CW, Dormitzer PR, Carfi A: Structural basis for immunization with postfusion respiratory syncytial virus fusion F glycoprotein (RSV F) to elicit high neutralizing antibody titers. Proc Natl Acad Sci 2011, 108(23): 9619-9624.
- Novak J, Potemkin VA: A new glimpse on the active site of SARS-CoV-2 3CLpro, coupled with drug repurposing study. Mol Divers 2022, 26(5): 2631-2645.
- Arabyan E, Kotsynyan A, Hakobyan A, Zakaryan H: Antiviral agents against African swine fever virus. Virus Res 2019, 270: 197669.
- Wang Y, Moon A, Huang J, Sun Y, Qiu HJ: Antiviral effects and underlying mechanisms of probiotics as promising antivirals. Front Cell Infect Microbiol 2022, 12: 928050.
- Agut H: Antivirales (a excepción del virus de la inmunodeficiencia humana y la hepatitis). EMC-Tratado Med 2022, 26(2): 1-10.
- Lee MF, Wu YS, Poh CL: Molecular mechanisms of antiviral agents against dengue virus. Viruses 2023, 15(3): 705.
- Geraghty RJ, Aliota MT, Bonnac LF: Broad-spectrum antiviral strategies and nucleoside analogues. Viruses 2021, 13(4): 667.
- Palacios-Rápalo SN, Farfan-Morales CN, Cordero-Rivera CD, De Jesús-González LA, Reyes-Ruiz JM, Meraz-Ríos MA, Del Ángel RM: An ivermectin–atorvastatin combination impairs nuclear transport inhibiting dengue infection in vitro and in vivo. IScience 2023, 26(12): 108294.
- Peng S, Wang H, Wang Z, Wang Q: Progression of antiviral agents targeting viral polymerases. Molecules 2022, 27(21): 7370.
- Ventre K, Randolph A: Ribavirin for respiratory syncytial virus infection of the lower respiratory tract in infants and young children. Cochrane Database Sys Rev 2007, (1): CD000181.
- Hoover J, Eades S, Lam WM: Pediatric Antiviral Stewardship: Defining the Potential Role of Ribavirin in Respiratory Syncytial Virus–Associated Lower Respiratory Illness. J Pediatr Pharmacol Ther 2018, 23(5): 372-378.
- Boeckh M, Englund J, Li Y, Miller C, Cross A, Fernandez H, Kuypers J, Kim H, Gnann J, Whitley R et al: NIAID Collaborative Antiviral Study Group. Randomized controlled multicenter trial of aerosolized ribavirin for respiratory syncytial virus upper respiratory tract infection in hematopoietic cell transplant recipients. Clin Infect Dis 2007, 44(2): 245-249.
- Sinclair SM, Jones JK, Miller RK, Greene MF, Kwo PY, Maddrey WC: The Ribavirin Pregnancy Registry: an interim analysis of potential teratogenicity at the mid-point of enrollment. Drug Saf 2017, 40(12): 1205-1218.
- Burrows FS, Carlos LM, Marriott D, Havryk A, Plit M, Glanville AR: Oral ribavirin is a cost-effective alternative to intravenous ribavirin for respiratory syncytial virus (RSV) infection after lung transplantation (LTx). J Heart Lung Transplant 2013, 34(7): 958-962.
- Tejada S, Martinez-Reviejo R, Karakoc HN, Peña-López Y, Manuel O, Rello J: Ribavirin for treatment of subjects with respiratory syncytial virus-related infection: a systematic review and meta-analysis. Adv Ther 2022, 39(9): 4037-4051.
- Med BNL: Identifier: NCT04225897. A Study in Infants With RSV LRTI to Evaluate RV521 (REVIRAL 1). 2020. Available online: https://www.clinicaltrials.gov/ct2/show/NCT04225897 (accessed on 29 November 2022).
- Med BNL: Identifier: NCT04231968. A Study of AK0529 in Chinese Infants Hospitalized With RSV (AirFLO). 2020. Available online: https://www.clinicaltrials.gov/ct2/show/NCT04231968 (accessed on 8 April 2022).
- Alansari K, Toaimah FH, Almatar DH, El Tatawy LA, Davidson BL, Qusad MI: Monoclonal antibody treatment of RSV bronchiolitis in young infants: a randomized trial. Randomized Controlled Trial 2019, 143(3): e20182308.
- Sáez-Llorens X, Moreno MT, Ramilo O, Sánchez PJ, Top Jr FH, Connor EM: MEDI-493 Study Group. Safety and pharmacokinetics of palivizumab therapy in children hospitalized with respiratory syncytial virus infection. Pediatr Infect Dis J 2004, 23(8): 707-712.
- Ramilo O, Lagos R, Sáez-Llorens X, Suzich J, Wang CK, Jensen KM, Harris BS, Losonsky GA, Griffin MP: Motavizumab Study Group. Motavizumab treatment of infants hospitalized with respiratory syncytial virus infection does not decrease viral load or severity of illness. Pediatr Infect Dis J 2014, 33(7): 703-709.
- Prince GA, Hemming VG, Horswood RL, Chanock RM: Immunoprophylaxis and immunotherapy of respiratory syncytial virus infection in the cotton rat. Virus Res 1985, 3(3): 193-206.
- Sanders SL, Agwan S, Hassan M, Bont LJ, Venekamp RP: Immunoglobulin treatment for hospitalised infants and young children with respiratory syncytial virus infection. Cochrane Database Syst Rev 2023, 10(10): CD009417.
- Domachowske JB, Anderson EJ, Goldstein M: The future of respiratory syncytial virus disease prevention and treatment. Infect Dis Ther 2021, 10(Suppl 1): 47-60.
- Hislop AA, Haworth SG: Airway size and structure in the normal fetal and infant lung and the effect of premature delivery and artificial ventilation. Am Rev Respir Dis 1989, 140(6): 1717-1726.
- Aherne W, Bird T, Gardner PS, McQuillin J: Pathological changes in virus infections of the lower respiratory tract in children. J Clin Pathol 1970, 23(1): 7-18.
- Pickles RJ, DeVincenzo JP: Respiratory syncytial virus (RSV) and its propensity for causing bronchiolitis. J Pathol 2015, 235(2): 266-276.
- Johnson JE, Gonzales RA, Olson SJ, Wright PF, Graham BS: The histopathology of fatal untreated human respiratory syncytial virus infection. Mod Pathol 2007, 20(1): 108-119.
- Larkin EK, Hartert TV: Genes associated with RSV lower respiratory tract infection and asthma: the application of genetic epidemiological methods to understand causality. Future Virol 2015, 10(7): 883-897.
- Shilovskiy IP, Yumashev KV, Nikolsky AA, Vishnyakova LI, Khaitov MR: Molecular and cellular mechanisms of respiratory syncytial viral infection: using murine models to understand human pathology. Biochemistry 2021, 86(3): 290-306.
- Linssen RS, Ma J, Bem RA, Rubin BK: Rational use of mucoactive medications to treat pediatric airway disease. Paediatr Respir Rev 2020, 36: 8-14.
- Kellner JD, Ohlsson A, Gadomski AM, Wang EE: Bronchodilators for bronchiolitis. Cochrane Database Syst Rev 2000, 2: CD001266.
- Krilov LR: Respiratory syncytial virus disease: update on treatment and prevention. Expert Rev Antiinfect Ther 2011, 9(1): 27-32.
- Merkus PJ, de Hoog M, Van Gent R, De Jongste JC: DNase treatment for atelectasis in infants with severe respiratory syncytial virus bronchiolitis. Eur Respir J 2001, 18(4): 734-737.
- Thornby KA, Johnson A, Axtell S: Dornase Alfa for Non–Cystic Fibrosis Pediatric Pulmonary Atelectasis. Ann Pharm 2014, 48(8): 1040-1049.
- Boogaard R, Hulsmann AR, Van Veen L, Vaessen-Verberne AA, Yap YN, Sprij AJ, Brinkhorst G, Sibbles B, Hendriks T, Feith SW et al: Recombinant human deoxyribonuclease in infants with respiratory syncytial virus bronchiolitis. Chest. 2007, 131(3): 788-795.
- Enriquez A, Chu IW, Mellis C, Lin WY: Nebulised deoxyribonuclease for viral bronchiolitis in children younger than 24 months. Cochrane Database Syst Rev 2012, 11(11): CD008395.
- Sathe NA, Krishnaswami S, Andrews J, Ficzere C, McPheeters ML: Pharmacologic agents that promote airway clearance in hospitalized subjects: a systematic review. Respir Care 2015, 60(7): 1061-1070.
- Mata M, Morcillo E, Gimeno C, Cortijo J: N-acetyl-L-cysteine (NAC) inhibit mucin synthesis and pro-inflammatory mediators in alveolar type II epithelial cells infected with influenza virus A and B and with respiratory syncytial virus (RSV). Biochem Pharmacol 2011, 82(5): 548-555.
- Naz F, Raza AB, Ijaz I, Kazi MY: Effectiveness of nebulized N-acetylcysteine solution in children with acute bronchiolitis. J Coll Physicians Surg Pak 2014, 24(6): 408-411.
- Wohl ME, Chernick V: State of the art: bronchiolitis. Am Rev Respir Dis 1978, 118(4): 759-781.
- Mandelberg A, Tal G, Witzling M, Someck E, Houri S, Balin A, Priel IE: Nebulized 3% hypertonic saline solution treatment in hospitalized infants with viral bronchiolitis. Chest 2003, 123(2): 481-487.
- Faber TE, Kamps AW, Sjoerdsma MH, Vermeulen S, Veeger NJ, Bont LJ: Computerized assessment of wheezing in children with respiratory syncytial virus bronchiolitis before and after hypertonic saline nebulization. Respir Care 2015, 60(9): 1252-1256.
- Zhang L, Gunther CB, Franco OS, Klassen TP: Impact of hypertonic saline on hospitalization rate in infants with acute bronchiolitis: A meta‐analysis. Pediatr Pulmonol 2018, 53(8): 1089-1095.
- Jaquet-Pilloud R, Verga ME, Russo M, Gehri M, Pauchard JY: Nebulised hypertonic saline in moderate-to-severe bronchiolitis: a randomised clinical trial. Arch Dis Child 2020, 105(3): 236-240.
- Kellner JD, Ohlsson A, Gadomski AM, Wang EE: Efficacy of bronchodilator therapy in bronchiolitis: a meta-analysis. Arch Pediatr Adolesc Med 1996, 150(11): 1166-1172.
- Rodríguez-Martínez CE, Nino G, Castro-Rodriguez JA, Acuña-Cordero R, Sossa-Briceño MP, Midulla F: For which infants with viral bronchiolitis could it be deemed appropriate to use albuterol, at least on a therapeutic trial basis? Allergol Immunopathol 2021, 49(1): 153-158.
- Sanchez I, De Koster J, Powell RE, Wolstein R, Chernick V: Effect of racemic epinephrine and salbutamol on clinical score and pulmonary mechanics in infants with bronchiolitis. J Pediatr 1993, 122(1): 145-151.
- Hartling L, Bialy LM, Vandermeer B, Tjosvold L, Johnson DW, Plint AC, Klassen TP, Patel H, Fernandes RM: Epinephrine for bronchiolitis. Cochrane Database Syst Rev 2011, 15(6): CD003123.
- Somers CC, Ahmad N, Mejias A, Buckingham SC, Carubelli C, Katz K, Leos N, Gomez AM, DeVincenzo JP, Ramilo O et al: Effect of dexamethasone on respiratory syncytial virus‐induced lung inflammation in children: results of a randomized, placebo controlled clinical trial. Pediatr Allergy Immunol 2009, 20(5): 477-485.
- Fernandes RM, Hartling L: Glucocorticoids for acute viral bronchiolitis in infants and young children. JAMA 2014, 311(1): 87-88.
- Zomer-Kooijker K, van der Ent CK, Ermers MJ, Rovers MM, Bont LJ: RSV Corticosteroid Study Group. Lack of long-term effects of high-dose inhaled beclomethasone for respiratory syncytial virus bronchiolitis: a randomized placebo-controlled trial. Pediatr Infect Dis J 2014, 33(1): 19-23.
- O'Donnell SR: Leukotrienes-biosynthesis and mechanisms of action. Aust Prescr 1999, 22(3): 55-57.
- Liu F, Ouyang J, Sharma AN, Liu S, Yang B, Xiong W, Xu R: Leukotriene inhibitors for bronchiolitis in infants and young children. Cochrane Database Syst Rev 2015, (3): CD010636.
- Tal G, Cesar K, Oron A, Houri S, Ballin A, Mandelberg A: Hypertonic saline/epinephrine treatment in hospitalized infants with viral bronchiolitis reduces hospitalization stay: 2 years experience. Isr Med Assoc J 2006, 8(3): 169-173.
- Flores-González JC, Matamala-Morillo MA, Rodríguez-Campoy P, Pérez-Guerrero JJ, Serrano-Moyano B, Comino-Vazquez P, Palma-Zambrano E, Bulo-Concellón R, Santos-Sánchez V, Lechuga-Sancho AM et al: Bronchiolitis of Cadiz Study group (BronCaS). Epinephrine improves the efficacy of nebulized hypertonic saline in moderate bronchiolitis: a randomised clinical trial. PLoS One 2015, 10(11): e0142847.
- Florin TA, Plint AC, Zorc JJ: Viral bronchiolitis. Lancet 2017, 389(10065): 211-224.
- Elliott SA, Gaudet LA, Fernandes RM, Vandermeer B, Freedman SB, Johnson DW, Plint AC, Klassen TP, Allain D, Hartling L et al: Comparative efficacy of bronchiolitis interventions in acute care: a network meta-analysis. Pediatrics 2021, 147(5): e2020040816.
- Balsamo R, Lanata L, Egan CG: Mucoactive drugs. Eur Respir Rev 2010, 19(116): 127-133.
- Roqué-Figuls M, Gine-Garriga M, Rugeles CG, Perrotta C, Vilaró J: Chest physiotherapy for acute bronchiolitis in paediatric patients between 0 and 24 months old. Cochrane Database Syst Rev 2023, 4(4): CD004873.
Asia-Pacific Journal of Pharmacotherapy & Toxicology
p-ISSN: 2788-6840
e-ISSN: 2788-6859
Copyright © Asia Pac J Pharmacother Toxicol. This work is licensed under a Creative Commons Attribution-NonCommercial-No Derivatives 4.0 International (CC BY-NC-ND 4.0) License.