Review Article | Open Access
Harnessing exosomes: the future of drug delivery across biological barriers
Rahul Choudhury1
1Department of Pharmacology, BCDA College of Pharmacy & Technology, Hridaypur, Barasat, India.
Correspondence: Rahul Choudhury (Department of Pharmacology, BCDA College of Pharmacy & Technology, Hridaypur, Barasat, India; E-mail: choudhury7rahul@gmail.com).
Asia-Pacific Journal of Pharmacotherapy & Toxicology 2025, 5: 1-10. https://doi.org/10.32948/ajpt.2025.01.10
Received: 16 Nov 2024 | Accepted: 19 Jan 2025 | Published online: 26 Jan 2025
Key words exosomes, drug delivery, biogenesis, isolation, drug loading tools
EVs were mainly unknown until the 1980s, when their secretion was recognized as a common cellular function, notwithstanding these early findings. In the 1980s, exosome research became popular, especially as a result of Trams et al.'s ectoenzyme studies [5]. Exosomes were also discovered in the seminal fluid around this time, and it was hypothesized that they came from the prostate and facilitated the transfer of proteins and lipids that were necessary for sperm maturation [6]. All prokaryotic and eukaryotic cell types secrete EVs, which are now characterized as membrane-bound vesicles with micro- or nano-scale dimensions [7]. EVs are classified as exosomes (30–150 nm), microvesicles (MVs) (100–1000 nm), and apoptotic bodies (>1000 nm) according to their shape and generation methods (Figure 1) [8]. Numerous components found in these vesicles mediate intercellular communication and impact a variety of cellular functions [9].
The processes of biogenesis distinguish apoptotic bodies, MVs, and exosomes [10]. MVs are created by the budding and blebbing of the cell membrane, exosomes come from endocytic pathways, and cells going through programmed cell death produce apoptotic bodies, which act as cues for phagocytic clearance. Exosomes are sometimes referred to as nanosomes and these vesicles are a promising platform for the development of targeted drug delivery systems to deliver therapeutic payloads to particular cells, tissues, or organs since they can transfer intracellular components and functional molecules to destination cells [11].
Exosomes are commonly found in a variety of biological fluids, such as urine, saliva, serum, and cerebrospinal fluid (CSF), as well as a broad spectrum of cell types, such as platelets and immune cells. According to preliminary studies, exosomes have been linked to several biological activities, including apoptosis, coagulation, inflammation, and maintaining cellular homeostasis [12]. Exosomes are lipid bilayer-encased, nanoscale vesicles that contain proteins, RNA, and other bioactive materials that facilitate intercellular communication. Their potential uses include serving as therapeutic tools and biomarkers for the identification and management of different illnesses [13].
The endosomal process, which starts with the bio-membrane budding inward and creates endosomes-membrane-bound vesicles inside cells-is how exosomes are produced [14]. These EVs transport biological signals, including transferrin receptors and transmembrane proteins, from their host cells to the outside world [15]. Exosomes engage with destination cells and transfer their molecular payload via this process [16]. Exosomes are important for intercellular communication because they carry proteins and RNA, which allows signals to be sent between cells and even distant organs [17]. Through receptor-ligand interactions, they interact with cell membranes and take part in several events, including the presentation of antigens and the advancement of cancer. Exosomes discharge their internal contents and surface proteins into the destination cell after attaching themselves to the target cell membrane. Exosomes have been shown to enter cells, carry their cargo, and alter different physiological and pathological functions [18].
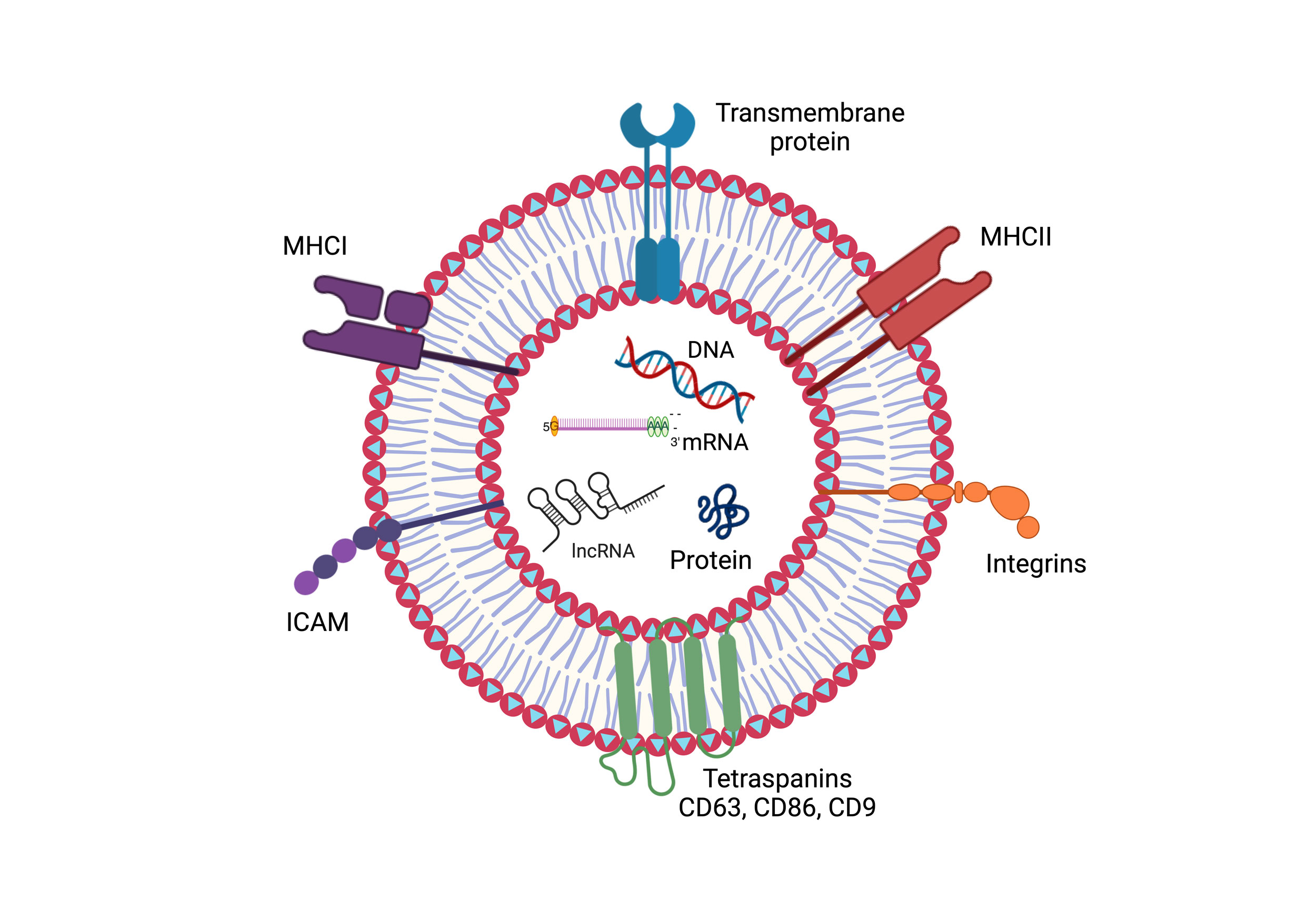
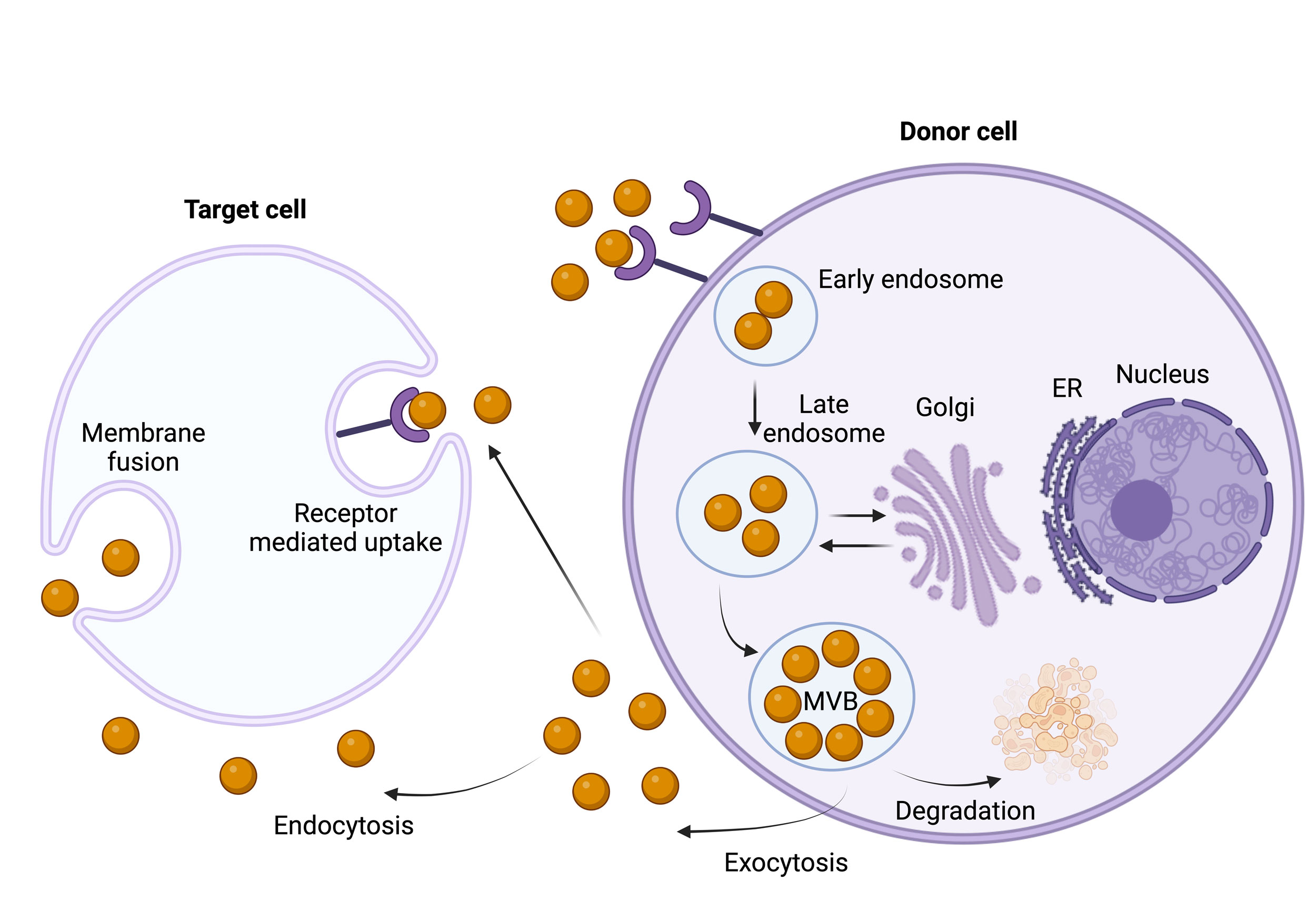
Ultrafiltration
The method of exosome separation is simple, size-dependent, and useful [26]. The benefit of ultracentrifugation is that it only needs a small sample volume to provide the required yield while being slower, more complicated, and less effective. The remarkable effectiveness of ultrafiltration was established by Cheruvanky et al., who presented that exosomes could be successfully separated using only 0.5 mL of a urine sample [27]. Techniques like tangential flow filtration and direct flow filtration are used in ultrafiltration. A common method for filtering tiny sample amounts (up to 30 mL) is direct flow filtration. However, issues including membrane fouling and insufficient particle separation limit this approach. Tangential flow filtration is a more potent and useful substitute for large-scale exosome isolation. Tangential flow filtration reduces clogging and stops membrane fouling layers from forming by guiding the sample stream across the ultrafilter membrane [28].
Immunological separation
Another technique for purifying exosomes is immunoaffinity. This method allows for more precise exosome isolation by using different antibodies. It is possible to apply these antibodies separately or in combination. Numerous substrates, including magnetic beads, chromatography matrices, plates, and microfluidic devices, can be used to immobilize the antibodies [29]. Although this approach is scalable, many of the extracellular antigens linked to exosomes are not unique to them. Therefore, protein complexes and other particles, including antigen-carrying vesicles unrelated to exosomes, such as cell debris, may also be purified using immunoaffinity-based isolation approaches. Additionally, this method may limit exosome isolation to those associated with a specific biomarker [30].
Ultracentrifugation
Ultracentrifugation is widely regarded as the conventional and benchmark technique for isolating exosomes. This technique relies on centrifugal force to separate cells and major cellular debris from biological fluids based on density, size, and shape [31]. Théry et al. outlined a detailed experimental protocol for exosome extraction and separation using ultracentrifugation. As centrifugation technologies continue to advance, the use of density gradient separation in combination with ultracentrifugation can further enhance exosome isolation [32]. However, despite its established effectiveness, prolonged application of ultracentrifugation may lead to the rupture of exosome membranes due to the sustained effects of centrifugal force [33].
Size exclusion chromatography
By trapping smaller particles in a column full of porous beads, size exclusion chromatography (SEC) is a chromatographic method that filters out particles according to their size. Larger particles, on the other hand, elute more rapidly. Exosomes are larger than most proteins, which usually have diameters of less than 10 nm. They can range in size from 40 to 100 nm. Using beads of the right size, centrifugation can be used to separate exosomes from proteins, leaving behind bigger cellular detritus. With pore diameters of 42, 31, and 75 nm, respectively, Sepharose CL-4B, Sephacryl S-400, and Sepharose CL-2B beads have been used to successfully separate exosomes from proteins [34]. SEC offers several benefits. It preserves the vesicular structure of exosomes, as they are not subjected to harsh conditions like centrifugal forces [35]. Due to its relatively low cost and rapid separation process, SEC is regarded as a practical method for large-scale exosome development.
Polymer-based precipitation separation
The current technique uses hydrophilic polymers, such as polyethylene glycol (PEG), to precipitate exosomes based on charge. By attaching themselves to water molecules, PEG decreases the solubility of exosomes, resulting in their precipitation at comparatively low centrifugal forces. Following an overnight incubation period at 4°C, exosome-containing samples that are treated with a PEG solution precipitate. Filtration or centrifugation can then be used to recover or isolate the precipitated exosomes. This method is comparatively easy to use, requires little downtime, and doesn't require sophisticated equipment [36].
Magnetic separation
Clayton et al. used immuno-magnetic isolation of exosomes expressing human primary histocompatibility complex class II molecules to suggest a quick and effective technique for routine exosome separation and exploration [30]. This technique makes use of magnetic beads coated with antibodies that are particular to the proteins found on the surface of exosomes. There are numerous benefits linked to this strategy. For example, the bead-exosome complexes can be analyzed using flow cytometry in conjunction with fluorophore-conjugated antibodies, allowing for a rapid and quantitative evaluation of the exosome surface composition [37].
Dielectrophoretic separation
The dielectric force acting on polarized particles in a non-uniform electric field is the basis for DEP separation. Larger objects like cells are drawn to the low-field zones between the electrodes, whereas nanoscale particles are drawn to the high-field zones close to the edges of circular microelectrodes. However, the DEP field has little influence on cations, anions, and small biomolecules. The dielectric characteristics of the surrounding fluid and the nanoparticles differ, which results in the DEP force [38]. The rate at which charges flow through a material in response to variations in the external electric field is determined by the dielectric constant. Transient dipoles are created across the nanoparticles as the fluid's charges and the nanoparticles' realignment rates diverge. The forces produced by these dipoles draw the nanoparticles into the non-uniform electric field's DEP high-field zones [39]. Although the technique's use may be restricted by electrothermal heating, its efficiency and speed are significant benefits.
Microfluidic devices
Even with small sample volumes, exosome separation and purification can be automated thanks to microfluidic devices [40]. EVs and their subtypes can be quickly and precisely purified with these technologies. These devices combine features including sieving barriers, mixing chambers, and antibody-coated functionalized layers to make it easier to separate various EV subclasses. The majority of these separation techniques rely on affinity [41]. Because microfluidic devices can handle small volumes (µL), they are very helpful as diagnostic instruments. They can, for example, enhance cell-specific EVs associated with particular illnesses. While they show great promise in diagnostics, these devices face significant challenges for large-scale applications due to issues such as the lack of standardization and the absence of consistent benchmarking, limiting their scalability in broader diagnostic contexts [42]. The principles, advantages, and disadvantages of different exosome isolation methods are summarized in the accompanying Table 1.
Table 1. Principles, advantages and disadvantages of exosome separation methods. |
||||
Method |
Principle |
Advantages |
Disadvantages |
Reference |
Ultrafiltration |
Separation is based on molecular weight and size |
Faster, requires no special equipment |
Exosome clogging, deformation, and damage to large EVs |
[66] |
Immunological Separation |
Exosomes are captured via an antigen-antibody response |
Saves time, isolates of high purity, and a simple process |
poor capacity, and yield, non-physiological salt and pH conditions are required |
[67] |
Ultracentrifugation |
Sedimentation coefficient of exosomes and other substances in the sample |
Exosomes may be produced in enormous quantities, with great separation purity |
Time required (>4 h), low recovery rate (5–25%), and poor reproducibility |
[66] |
Size-Exclusion Chromatography |
Utilizes a column of porous polymeric beads to separate exosomes based on size |
High yield and purity |
Expensive, time-consuming post-isolation analysis and column contamination |
[68] |
Polymer-based precipitation separation |
Hydrophobicity |
Simple procedure with a small sample volume |
Long run periods and post-separation cleaning are required |
[69] |
Magnetic separation |
Magnetic Force |
The contactless separation, high specificity, and high throughput |
Magnetic labeling |
[70] |
Dielectrophoretic separation
|
Polarized particle’s size and electric properties |
Label-free, contactless, fast, and high-throughput |
Low resolution, low purity, Joule and electrothermal heating problems |
[71] |
Microfluidic devices |
Separation based on size, charge, surface properties and interactions |
Fast, high precision |
Non-scalability on large-scale diagnostics |
[72] |
Incubation of drugs with exosomes
By incubating the drug with exosomes, this method often called passive drug loading allows the medication to diffuse into the exosomes following the concentration gradient. Given that hydrophobic medications are more likely to engage with the vesicle's lipid bilayer, the effectiveness of drug loading with this technique is directly correlated with the hydrophobicity of the drug molecules [44]. In one investigation, Dongmel et al. treated mouse lymphoma exosomes with curcumin in PBS for five minutes at 22°C. A sucrose gradient was then used to centrifuge the mixture. When compared to its free form, curcumin's solubility, stability, and bioavailability were all increased by this encapsulating technique [45]. Similarly, when curcumin was incubated with exosomes, Vashisht et al. [46] showed a 70.46% drug loading efficiency. Catalase was also encapsulated into exosomes by an 18-hour incubation period in PBS at room temperature. Nonetheless, this approach's comparatively poor drug-loading capacity is a major drawback [47].
Incubation of drugs with donor cells
This technique involves administering a medication to the target donor cells, which enables the cells to accumulate therapeutic or bioactive substances. The therapeutic chemicals can then be transported by the exosomes secreted by these pretreated cells. This method aims to deliver the therapeutic compounds into the exosomes after they have been absorbed and packaged by the donor cells. However, this strategy can result in a reduced exosome yield because it is untargeted [44]. A modest dose of paclitaxel was administered to SR4987 mesenchymal stromal cells for 24 hours in a study conducted by Pascucci et al. [48]. Following a wash, the cells were moved to a fresh culture flask containing new media. The paclitaxel-loaded exosomes were separated from the conditioned media after 48 hours of culture. To promote the release of drug-loaded exosomes, donor cells can also undergo mechanical or biological treatments, such as exposure to heat, UV light, or a combination of these [40, 49].
Active drug loading approaches
By momentarily rupturing the exosome membrane, active drug loading facilitates the drug's easier diffusion into the vesicles. The exosome membrane is repaired to preserve its integrity after the intended payload has been loaded. The exosome membrane can be broken by a variety of techniques, such as sonication, extrusion, and freeze-thaw cycles [43]. The active approach can boost the drug-loading capacity of exosome vesicles by up to 11 times compared to passive drug loading [50]. The possibility of harming the exosome's original structure and targeting capabilities during the disruption process is a significant obstacle to this strategy, but [43].
Sonication
After combining the medication or protein of interest with exosomes generated from donor or target cells, a homogenizer probe is employed to sonicate the mixture. The exosome membrane is broken by the mechanical shear force which also deforms the membrane and permits the diffusion of bioactive substances into the vesicle [51]. After sonication, Kim et al. [52] found that the exosome membrane's microviscosity significantly decreased. However, the exosome's lipid contents and membrane-bound proteins are not significantly impacted by this deformation procedure. They discovered that an hour of incubation at 37°C might restore the integrity of the exosome membrane. Furthermore, when medications are both contained inside and connected to the outside membrane of exosomes, biphasic drug release may occasionally happen. A burst release occurs when the medication attaches to the outside membrane of the exosome, whereas a gradual release occurs when the drug is encapsulated inside [52].
Extrusion
Extrusion is a post loading technique that loads medications into exosomes using a lipid extruder based on a syringe. Donor cell exosomes are combined with a target medication and put into a syringe-based lipid extruder, which is kept at a regulated temperature and contains a membrane with pores that range in size from 100 to 400 nm. The medication and the damaged exosome membrane are well combined during extrusion [53]. The benefits of using the extrusion technique to load medications into exosomes were emphasized by Fuhrmann et al. [50]. Porphyrin was extruded into exosomes made from MDA-MB231 breast cancer cells for their investigation. When compared to the incubation procedure, this method produced a higher cytotoxic effect. Furthermore, the extrusion process modifies the exosomes' zeta potential, and by changing the makeup of the vesicles, increasing the number of extrusions during an intense extrusion procedure can improve drug loading even more.
Freeze-thaw cycles
Exosomes are first incubated with the target drug at ambient temperature for a certain amount of time, and then they are quickly frozen at -80 °C or in liquid nitrogen as part of the freeze-thaw process for drug loading. After that, the mixture is allowed to defrost at room temperature. Freeze-thaw cycles are usually performed at least three times to improve medication encapsulation. However, in comparison to sonication or extrusion procedures, this technique often has a lesser drug-loading potential. Furthermore, it might result in exosome aggregation, which would cause the drug-loaded exosomes to have a larger size distribution [54].
Electroporation
Through the disruption of the phospholipid bilayer and the creation of pores in it, electroporation uses an electromagnetic field to encourage the passage of medicinal molecules into the exosome lumen [55]. Drug molecules permeate the exosome membrane's pores during this process, and following loading, the membrane's integrity is restored. This method is frequently used to load big molecules into exosomes, like nucleotides (like siRNA or miRNA) [50]. However, because of problems such as RNA aggregation and exosome instability, electroporation often has a reduced loading capacity. Despite this, the technique can increase the incorporation of hydrophilic small molecules into exosomes and substantially raise RNA loading [50].
Incubation with membrane permeabilizers
The cholesterol in the cell membrane interacts with membrane permeabilizers and surfactants, including saponin, to create pores that make the exosomal membrane more permeable. The membrane permeability technique can increase the effectiveness of catalase loading into exosomes in comparison to the incubation approach [56]. In comparison to the passive loading technique without saponin, a previous study showed that the use of saponin increased the loading of hydrophilic medicines into exosomes by 11-fold [50]. Using the right amount of saponin for drug loading is crucial in this method, and purification of the exosomes is necessary following the saponin incubation.
Table 2. Advantages and disadvantages of different exosome drug loading approaches. |
|||
Drug loading approach |
Principle |
Advantages |
Disadvantages |
Incubation of exosomes and free drugs/ Incubation of the donor cells with free drugs
|
Diffusion of cargo into a cell or exosomal membrane |
Simple operation |
Loading efficiency. Drugs may cause cytotoxicity to the donor cells |
Sonication |
Creation of micropores for diffusion by mechanical shear force |
Higher loading capacity than the simple incubation method |
Sonication-induced membrane damage is a roadblock for large-scale application |
Extrusion |
Membrane recombination |
High cargo loading efficiency. Repeated extrusion provides a homogeneous blend of exosomes with cargoe |
Recombination of exosomal surface structure may compromise the immune-privileged status of exosomes, making exosomes visible to immune cells such as mononuclear phagocytes |
Freeze-thaw cycles |
Membrane fusion |
A simple and effective strategy to load various cargoes into exosomes directly |
Repeated freeze-thaw may cause protein degeneration and exosome aggregation |
Electroporation |
Creation of micropores for diffusion by the electric field |
High loading efficiency |
The loading efficiency and aggregation of cargo are major limitations |
Incubation with membrane permeabilizes |
Dissolves membrane molecules, creates pores on the exosomal surface |
Higher loading capacity as compared with the simple incubation method |
An extra purification process may be required to remove saponin |
Note: Reference (47, 56). |
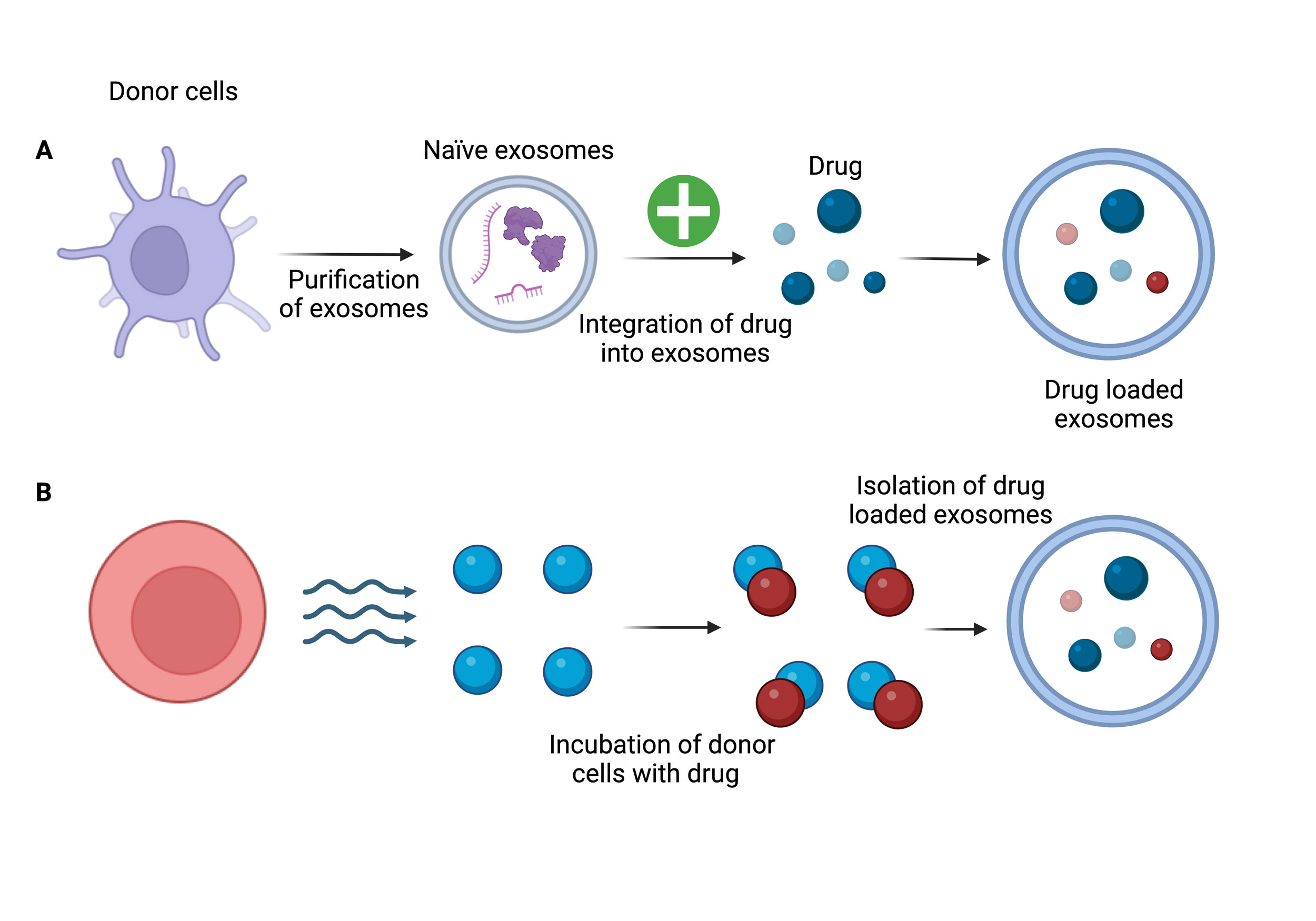
ExoSTING, an antigen-presenting cell-targeting agent created by Codiak, distributes interferon gene stimulators within the lumen of exosomes. It has been demonstrated that this drug maintains memory responses against tumor cells. Furthermore, Codiak has created an exosome product called exoASOTM-STAT6 that specifically targets tumor macrophages [58]. Exosomal topical solution for diabetic ulcers and chronic wounds, as well as exosomal eye serum for glaucoma and age-related macular degeneration, are developments for Vittilabs. Exosomes have been shown in numerous studies to be effective ocular pharmacotherapeutics for a variety of diseases [59].
To enable tailored delivery to particular organs, the Evox Therapeutics scientific team is working on creating protein engineering methods that load proteins and nucleic acids into exosomes. Along with implementing trade secrets, copyrights, and trademarks to safeguard its assets, Evox has also developed a portfolio of intellectual property that includes its exosome drug delivery systems (DDS) for mRNA, siRNA, antibodies, receptors, antisense oligonucleotides, enzyme replacement therapies, and CRISPR technologies. Evox also possesses proprietary technology for downstream processing, upstream process optimization, and cellular engineering. Other top businesses, like XOSTEM, TEVAC Pharmaceuticals, OmniSpirant, and ILIAS, are also attempting to resolve the manufacturing and engineering issues required for the repeatable production of transformational exosome medications. Bone diseases, fibrotic conditions, alopecia, heart diseases, skin problems, gastrointestinal disorders, kidney diseases, rare genetic disorders, autism spectrum disorder, neurodegenerative diseases, cancer, diabetes, wound healing, and transplant rejection are just a few of the many illnesses for which exosomes are being studied [57]. The thorough analysis of exosomes highlights that to better understand the diverse ways that exosomes respond to communication between normal and malignant cells, future research should concentrate on filling in the gaps that currently exist, especially in vivo studies. Deciphering exosomal transport pathways and target cell physiological regulation are part of this. Furthermore, to fully evaluate toxicity and direct the therapeutic potential of exosomes, more methodical in vivo research is required. These new nanoplatforms may become closer to clinical use as a result of these investigations. Even at the preclinical level, it is critical to choose and create suitable animal models that also satisfy economic sustainability, even if exosome clinical trials are still in their infancy and have not yet addressed numerous clinical transition issues. In this regard, organ-on-a-chip systems may be a strong and trustworthy instrument for investigating the efficacy, drug delivery potential, and mechanisms of action of exosomes [60].
Animals are not the only things that have exosomes; plants and plant-derived products, such as fruit juices, have also been found to contain them. Grape exosomes, for instance, have demonstrated potential in the treatment of radiation or chemotherapy-induced oral mucositis [61]. Exosomes generated from grapes have been shown in preclinical research to contribute to tissue remodeling following pathological injury and support intestinal tissue regeneration [62]. To treat patients with polycystic ovarian syndrome and lessen insulin resistance and chronic inflammation, another clinical trial is examining the effects of exosomes made from ginger or aloe (NCT03493984). Furthermore, because curcumin is hydrophobic, Donar Millar et al. investigated the utilization of plant-derived exosomes as a hydrophobic drug delivery method to encapsulate the molecule for the treatment of intestinal diseases [63]. To make the exosome isolation procedure simpler, more studies should concentrate on sources that don't include cells or animals.
It has been noted that mesenchymal stem cells or other stem cell types are the source of the majority of exosomes that make it to human clinical trials. To address the increasing demands of cutting-edge research, biobanks devoted to the preservation of stem cells have grown significantly on a global scale. In light of this growing demand, the discipline of biobanking emphasizes the exosome's collection, storage, and dissemination, in addition to the exosome's place of origin. Addressing information gaps about specific processes, transport variables, isolation yields, preclinical parameters, and other elements that are frequently underreported in the literature requires an understanding of the exosome lifecycle. To overcome the present obstacles in exosome research, biobank operations are crucial in bridging the gap between bench research and clinical applications [64]. To guarantee the quality of exosomes in biobanks, however, additional standardized techniques, regulated processes, and accurate data archiving are urgently needed. Monitoring negative reactions when exosomes are released onto the market is equally crucial [65].
No applicable.
Ethics approval
No applicable.
Data availability
The data will be available upon request.
Funding
None.
Authors’ contribution
Rahul Choudhury contributed to draft, critical revision of the article, table making, figure production and submitted the final manuscript.
Competing interests
None.
- Anderson HC: Vesicles associated with calcification in the matrix of epiphyseal cartilage. J Cell Biol 1969, 41(1): 59-72.
- Wolf P: The nature and significance of platelet products in human plasma. Br J Haematol 1967, 13(3): 269-288.
- Pan BT, Teng K, Wu C, Adam M, Johnstone RM: Electron microscopic evidence for externalization of the transferrin receptor in vesicular form in sheep reticulocytes. J Cell Biol 1985, 101(3): 942-948.
- Bakhshian Nik A, Hutcheson JD, Aikawa E: Extracellular vesicles as mediators of cardiovascular calcification. Front Cardiovas Med 2017, 4: 78.
- Trams EG, Lauter CJ, Salem JN, Heine U: Exfoliation of membrane ecto-enzymes in the form of micro-vesicles. Biochim Biophys Acta 1981, 645(1): 63-70.
- Arienti G, Carlini E, Verdacchi R, Cosmi EV, Palmerini CA: Prostasome to sperm transfer of CD13/aminopeptidase N (EC 3.4. 11.2). Biochim Biophys Acta 1997, 1336(3): 533-538.
- Woith E, Fuhrmann G, Melzig MF: Extracellular vesicles-connecting kingdoms. Int J Mol Sci 2019, 20(22): 5695.
- Buschmann D, Mussack V, Byrd JB: Separation, characterization, and standardization of extracellular vesicles for drug delivery applications. Adv Drug Deliv Rev 2021, 174: 348-368.
- Habib A, Liang Y, Zhu N: Exosomes multifunctional roles in HIV-1: insight into the immune regulation, vaccine development and current progress in the delivery system. Front Immunol 2023, 14: 1249133.
- Akers JC, Gonda D, Kim R, Carter BS, Chen CC: Biogenesis of extracellular vesicles (EV): exosomes, microvesicles, retrovirus-like vesicles, and apoptotic bodies. Neurooncol 2013, 113(1): 1-11.
- Chung IM, Rajakumar G, Venkidasamy B, Subramanian U, Thiruvengadam M: Exosomes: Current use and future applications. Clin Chim Acta 2020, 500: 226-232.
- Gurunathan S, Kang MH, Jeyaraj M, Qasim M, Kim JH: Review of the isolation, characterization, biological function, and multifarious therapeutic approaches of exosomes. Cells 2019, 8(4): 307.
- Araujo-Abad S, Saceda M, de Juan Romero C: Biomedical application of small extracellular vesicles in cancer treatment. Adv Drug Deliv Rev 2022, 182: 114117.
- Abels ER, Breakefield XO: Introduction to extracellular vesicles: biogenesis, RNA cargo selection, content, release, and uptake. Cell Mol Neurobiol 2016, 36(3): 301-312.
- Kowal J, Tkach M, Théry C: Biogenesis and secretion of exosomes. Curr Opin Cell biol 2014, 29: 116-125.
- Braccioli L, Van Velthoven C, Heijnen CJ: Exosomes: a new weapon to treat the central nervous system. Mol Neurobiol 2014, 49(1): 113-119.
- Yuan D, Zhao Y, Banks WA, Bullock KM, Haney M, Batrakova E, Kabanov AV: Macrophage exosomes as natural nanocarriers for protein delivery to inflamed brain. Biomaterials 2017, 142: 1-12.
- Zhou Y, Tian T, Zhu Y, Jaffar Ali D, Hu F, Qi Y, Sun B, Xiao Z: Exosomes transfer among different species cells and mediating miRNAs delivery. J Cell Biochem 2017, 118(12): 4267-4274.
- Zhang Y, Bi J, Huang J, Tang Y, Du S, Li P: Exosome: a review of its classification, isolation techniques, storage, diagnostic and targeted therapy applications. Int J Nanomedicine 2020, 15: 6917-6934.
- Morelli AE, Larregina AT, Shufesky WJ, Sullivan ML, Stolz DB, Papworth GD, Zahorchak AF, Logar AJ, Wang Z, Watkins SC et al: Endocytosis, intracellular sorting, and processing of exosomes by dendritic cells. Blood 2004, 104(10): 3257-3266.
- Patil SM, Sawant SS, Kunda NK: Exosomes as drug delivery systems: A brief overview and progress update. Eur J Pharm Biopharm 2020, 154: 259-269.
- Abels ER, Breakefield XO: Introduction to extracellular vesicles: biogenesis, RNA cargo selection, content, release, and uptake. Cell Mol Neurobiol 2016, 36(3): 301-312.
- Kim H, Kim EH, Kwak G, Chi SG, Kim SH, Yang Y: Exosomes: cell-derived nanoplatforms for the delivery of cancer therapeutics. Int J Mol Sci 2020, 22(1): 14.
- Banerjee A, Jain SM, S Abrar S, Kumar MM, Mathew C, Pathak S: Sources, isolation strategies and therapeutic outcome of exosomes at a glance. Regen Med 2020, 15(12): 2361-2378.
- Blázquez R, Sánchez-Margallo FM, Álvarez V, Usón A, Marinaro F, Casado JG: Fibrin glue mesh fixation combined with mesenchymal stem cells or exosomes modulates the inflammatory reaction in a murine model of incisional hernia. Acta Biomater 2018, 71: 318-329.
- Cheruvanky A, Zhou H, Pisitkun T, Kopp JB, Knepper MA, Yuen PS, Star RA: Rapid isolation of urinary exosomal biomarkers using a nanomembrane ultrafiltration concentrator. Am J Physiol Renal Physiol 2007, 292(5): F1657-1661.
- Busatto S, Vilanilam G, Ticer T, Lin WL, Dickson DW, Shapiro S, Bergese P, Wolfram J: Tangential flow filtration for highly efficient concentration of extracellular vesicles from large volumes of fluid. Cells 2018, 7(12): 273.
- Chen C, Skog J, Hsu CH, Lessard RT, Balaj L, Wurdinger T, Carter BS, Breakefield XO, Toner M, Irimia D et al: Microfluidic isolation and transcriptome analysis of serum microvesicles. Lab Chip 2010, 10(4): 505-511.
- Théry C, Amigorena S, Raposo G, Clayton A: Isolation and characterization of exosomes from cell culture supernatants and biological fluids. Curr Protoc Cell Biol 2006, 30(1): 3-22.
- Greening DW, Xu R, Ji H, Tauro BJ, Simpson RJ: A protocol for exosome isolation and characterization: evaluation of ultracentrifugation, density-gradient separation, and immunoaffinity capture methods. Methods Mol Biol 2015, 1295: 179-209.
- Liao W, Du Y, Zhang C, Pan F, Yao Y, Zhang T, Peng Q: Exosomes: the next generation of endogenous nanomaterials for advanced drug delivery and therapy. Acta Biomater 2019, 86: 1-14.
- Lobb RJ, Becker M, Wen Wen S, Wong CS, Wiegmans AP, Leimgruber A, Möller A: Optimized exosome isolation protocol for cell culture supernatant and human plasma. J Extracell Vesicles 2015, 4(1): 27031.
- Baranyai T, Herczeg K, Onódi Z, Voszka I, Módos K, Marton N, Nagy G, Mäger I, Wood MJ, El Andaloussi S et al: Isolation of exosomes from blood plasma: qualitative and quantitative comparison of ultracentrifugation and size exclusion chromatography methods. PloS One 2015, 10(12): e0145686.
- Gámez-Valero A, Monguió-Tortajada M, Carreras-Planella L, Franquesa ML, Beyer K, Borràs FE: Size-Exclusion Chromatography-based isolation minimally alters Extracellular Vesicles’ characteristics compared to precipitating agents. Scientific Rep 2016, 6(1): 33641.
- Yang XX, Sun C, Wang L, Guo XL: New insight into isolation, identification techniques and medical applications of exosomesJ Control Release 2019, 308: 119-129.
- Zeringer E, Barta T, Li M, Vlassov AV: Strategies for isolation of exosomes. Cold Spring Harb Protoc 2015, 2015(4): 319-323.
- Clayton A, Court J, Navabi H, Adams M, Mason MD, Hobot JA, Newman GR, Jasani B: Analysis of antigen presenting cell derived exosomes, based on immuno-magnetic isolation and flow cytometry. J Immunol Methods 2001, 247(1-2): 163-174.
- Ibsen SD, Wright J, Lewis JM, Kim S, Ko SY, Ong J, Manouchehri S, Vyas A, Akers J, Chen CC et al: Rapid isolation and detection of exosomes and associated biomarkers from plasma. ACS Nano 2017, 11(7): 6641-6651.
- Huang LR, Cox EC, Austin RH, Sturm JC: Continuous particle separation through deterministic lateral displacement. Science 2004, 304(5673): 987-990.
- Contreras-Naranjo JC, Wu HJ, Ugaz VM: Microfluidics for exosome isolation and analysis: enabling liquid biopsy for personalized medicine. Lab Chip 2017, 17(21): 3558-3577.
- Lu B, Ku J, Flojo R, Olson C, Bengford D, Marriott G: Exosome-and extracellular vesicle-based approaches for the treatment of lysosomal storage disorders. Adv Drug Deliv Rev 2022, 188: 114465.
- Boriachek K, Islam MN, Möller A, Salomon C, Nguyen NT, Hossain MS, Yamauchi Y, Shiddiky MJ: Biological functions and current advances in isolation and detection strategies for exosome nanovesicles. Small 2018, 14(6): 1702153.
- Akuma P, Okagu OD, Udenigwe CC: Naturally occurring exosome vesicles as potential delivery vehicle for bioactive compounds. Front Sustain Food Syst 2019, 3: 23.
- Luan X, Sansanaphongpricha K, Myers I, Chen H, Yuan H, Sun D: Engineering exosomes as refined biological nanoplatforms for drug delivery. Acta Pharmacol Sin 2017, 38(6): 754-763.
- Sun D, Zhuang X, Xiang X, Liu Y, Zhang S, Liu C, Barnes S, Grizzle W, Miller D, Zhang HG et al: A novel nanoparticle drug delivery system: the anti-inflammatory activity of curcumin is enhanced when encapsulated in exosomes. Mol Ther 2010, 18(9): 1606-1614.
- Vashisht M, Rani P, Onteru SK, Singh D: Curcumin encapsulated in milk exosomes resists human digestion and possesses enhanced intestinal permeability in vitro. Appl Biochem Biotechnol 2017, 183(3): 993-1007.
- Haney MJ, Klyachko NL, Zhao Y, Gupta R, Plotnikova EG, He Z, Patel T, Piroyan A, Sokolsky M, Kabanov AV et al: Exosomes as drug delivery vehicles for Parkinson's disease therapy. J Control Release 2015, 207: 18-30.
- Pascucci L, Coccè V, Bonomi A, Ami D, Ceccarelli P, Ciusani E, Viganò L, Locatelli A, Sisto F, Doglia SM et al: Paclitaxel is incorporated by mesenchymal stromal cells and released in exosomes that inhibit in vitro tumor growth: a new approach for drug delivery. J Control Release 2014, 192: 262-270.
- Wang LY, Shi XY, Yang CS, Huang DM: Versatile RBC-derived vesicles as nanoparticle vector of photosensitizers for photodynamic therapy. Nanoscale 2013, 5(1): 416-421.
- Fuhrmann G, Serio A, Mazo M, Nair R, Stevens MM: Active loading into extracellular vesicles significantly improves the cellular uptake and photodynamic effect of porphyrins. J Control Release 2015, 205: 35-44.
- Veerabrahma K: Development of olmesartan medoxomil lipid-based nanoparticles and nanosuspension: preparation, characterization and comparative pharmacokinetic evaluation. Artif Cells Nanomed Biotechnol 2017, 46(1): 126-137.
- Kim MS, Haney MJ, Zhao Y, Mahajan V, Deygen I, Klyachko NL, Inskoe E, Piroyan A, Sokolsky M, Okolie O et al: Development of exosome-encapsulated paclitaxel to overcome MDR in cancer cells. Nanomedicine 2016, 12(3): 655-664.
- Kommineni N, Meckes D, Sachdeva M: Exosome vehicles as nano-drug delivery materials for chemotherapeutic drugs. Crit Rev Ther Drug Carrier Syst 2021, 38(5): 53-97.
- Sato YT, Umezaki K, Sawada S, Mukai SA, Sasaki Y, Harada N, Shiku H, Akiyoshi K: Engineering hybrid exosomes by membrane fusion with liposomes. Sci Rep 2016, 6(1): 21933.
- Johnsen KB, Gudbergsson JM, Skov MN, Pilgaard L, Moos T, Duroux M: A comprehensive overview of exosomes as drug delivery vehicles-endogenous nanocarriers for targeted cancer therapy. Biochim Biophys Acta 2014, 1846(1): 75-87.
- Podolak I, Galanty A, Sobolewska D: Saponins as cytotoxic agents: a review. Phytochem Rev 2010, 9(3): 425-474.
- Tenchov R, Sasso JM, Wang X, Liaw WS, Chen CA, Zhou QA: Exosomes-nature’s lipid nanoparticles, a rising star in drug delivery and diagnostics. ACS Nano 2022, 16(11): 17802-17846.
- Lee JY, Kim HS: Extracellular vesicles in regenerative medicine: potentials and challenges. Tissue Eng Regen Med 2021, 18(4): 479-484.
- Zhang Z, Mugisha A, Fransisca S, Liu Q, Xie P, Hu Z: Emerging role of exosomes in retinal diseases. Front Cell Develop Biol 2021, 9: 643680.
- Wu G, Wu J, Li Z, Shi S, Wu D, Wang X, Xu H, Liu H, Huang Y, Wang R et al: Development of digital organ-on-a-chip to assess hepatotoxicity and extracellular vesicle-based anti-liver cancer immunotherapy. Bio-Design Manufac 2022, 5(3): 437-450.
- Bruno SP, Paolini A, D'Oria V, Sarra A, Sennato S, Bordi F, Masotti A: Extracellular vesicles derived from citrus sinensis modulate inflammatory genes and tight junctions in a human model of intestinal epithelium. Front Nutr 2021, 8: 778998.
- Ju S, Mu J, Dokland T, Zhuang X, Wang Q, Jiang H, Xiang X, Deng ZB, Wang B, Zhang L et al: Grape exosome-like nanoparticles induce intestinal stem cells and protect mice from DSS-induced colitis. Mol Ther 2013, 21(7): 1345-1357.
- Chen P, Zheng L, Wang Y, Tao M, Xie Z, Xia C, Gu C, Chen J, Qiu P, Mei S et al: Desktop-stereolithography 3D printing of a radially oriented extracellular matrix/mesenchymal stem cell exosome bioink for osteochondral defect regeneration. Theranostics 2019, 9(9): 2439-2459.
- Mora EM, Álvarez-Cubela S, Oltra E: Biobanking of exosomes in the era of precision medicine: are we there yet?. Int J Mol Sci 2015, 17(1): 13.
- Nisa ZU, Zafar A, Zafar F, Pezaro S, Sher F: Adverse drug reaction monitoring and reporting among physicians and pharmacists in Pakistan: a cross-sectional study. Curr Drug Saf 2020, 15(2): 137-146.
- Li P, Kaslan M, Lee SH, Yao J, Gao Z: Progress in exosome isolation techniques. Theranostics 2017, 7(3): 789-804.
- Li Y, Zheng Q, Bao C, Li S, Guo W, Zhao J, Chen D, Gu J, He X, Huang S et al: Circular RNA is enriched and stable in exosomes: a promising biomarker for cancer diagnosis. Cell Res 2015, 25(8): 981-984.
- Chen BY, Sung CW, Chen C, Cheng CM, Lin DP, Huang CT, Hsu MY: Advances in exosomes technology. Clin Chim Acta 2019, 493: 14-19.
- Soares Martins T, Catita J, Martins Rosa I, AB da Cruz e Silva O, Henriques AG: Exosome isolation from distinct biofluids using precipitation and column-based approaches. PloS One 2018, 13(6): e0198820.
- Gámez-Valero A, Monguió-Tortajada M, Carreras-Planella L, Franquesa ML, Beyer K, Borràs FE: Size-Exclusion Chromatography-based isolation minimally alters Extracellular Vesicles’ characteristics compared to precipitating agents. Sci Rep 2016, 6(1): 33641.
- Tayebi M, Zhou Y, Tripathi P, Chandramohanadas R, Ai Y: Exosome purification and analysis using a facile microfluidic hydrodynamic trapping device. Anal Chem 2020, 92(15): 10733-10742.
- Boriachek K, Islam MN, Möller A, Salomon C, Nguyen NT, Hossain MS, Yamauchi Y, Shiddiky MJ: Biological functions and current advances in isolation and detection strategies for exosome nanovesicles. Small 2018, 14(6): 1702153.
Asia-Pacific Journal of Pharmacotherapy & Toxicology
p-ISSN: 2788-6840
e-ISSN: 2788-6859
Copyright © Asia Pac J Pharmacother Toxicol. This work is licensed under a Creative Commons Attribution-NonCommercial-No Derivatives 4.0 International (CC BY-NC-ND 4.0) License.