Review Article | Open Access
GLP-1 receptor agonism in cardiovascular disease prevention
Hussain Saleh1
1Department of Pharmacology, Pharmacy College, Karary University, Khartoum, Sudan.
Correspondence: Hussain Saleh (Department of Pharmacology, Pharmacy College, Karary University, Khartoum, Sudan; E-mail: Dr-Salehhussain@outlook.com).
Asia-Pacific Journal of Pharmacotherapy & Toxicology 2024, 4: 112-121. https://doi.org/10.32948/ajpt.2024.11.22
Received: 16 Sep 2024 | Accepted: 23 Nov 2024 | Published online: 30 Dec 2024
Key words GLP1, GLP-1RAs, cardiovascular disease, atherosclerosis, diabetes
In 2008, Food and Drug Administration (FDA) mandated that cardiovascular safety assessments are necessary for all new anti-diabetic drugs, leading to evaluation of all newly developed glucose-lowering therapies in cardiovascular outcome trials on regular basis [6]. In this context, glucagon-like peptide-1 receptor agonists (GLP-1RAs) were first identified for their effectiveness in diabetes management by mimicking the natural GLP-1 produced in the body. These agonists enhance insulin secretion and reduce glucagon levels; hence, effectively regulate blood glucose levels [7]. Alternatively, GLP-1RAs exhibit both direct and indirect cardioprotective properties, limiting the risk of major adverse cardiovascular event (MACE), particularly ischaemic one related to CVD [8]. Substantial preclinical and clinical research over the years corroborated the cardiovascular benefits of GLP-1RAs, which are primarily linked to their role in alleviating atherosclerosis by managing associated risk factors [9]. Here, we first discuss the molecular mechanisms through which GLP-1 and GLP-1RAs regulate insulin secretion and maintain glucose homeostasis. Later, we explore the role of GLP-1RAs in CVD prevention, emphasizing their direct and indirect impacts. Lastly, we highlight clinical advancements in the field and examine real-world data on their use in CVD management.
GLP-1RAs mimic endogenous GLP-1 and activates the GLP-1R, a type of G protein-coupled receptor (GPCR). Upon binding, GTP-bound Gαs stimulates adenylyl cyclase to convert ATP into cyclic AMP (cAMP). Protein kinase A (PKA), upon activation by cAMP, phosphorylates the sulfonylurea receptor-1 component of the K+/ATPase channel. This action accelerates the closure of the channel, facilitating an increased influx of calcium ions through voltage-gated calcium channels. The elevated calcium levels enhance insulin release through the Gq/phospholipase C pathway, where inositol trisphosphate (IP3) and diacylglycerol activate their respective receptors, the IP3 receptor (IP3R) and the ryanodine receptor, triggering calcium release (Figure 1) [11]. Additionally, Epac2, a cAMP-regulated exchange protein, activates ras-proximate-1 (Rap1), thereby enhancing phospholipase C (PLC) signaling-dependent calcium release [15]. The transient receptor potential melastatin 2 (TRPM2) channel plays an additional role in facilitating GLP-1-induced insulin secretion through pathways involving cAMP and PKA signaling [16]. To prevent desensitization, GLP-1R undergoes regular internalization by Gaq and β-arrestin pathways, enabling recycling and intracellular trafficking [17].
GLP-1RAs improve pancreatic β cell function as well, preventing their exhaustion by activating transcriptional pathways that promote proliferation, inhibit apoptosis, and optimize intracellular metabolism [18, 19]. Beyond pancreatic effects, GLP-1R agonists exert widespread extra-pancreatic benefits, attributed to the extensive distribution of GLP-1R in different organs, including gastrointestinal tract, kidneys, liver, skeletal and smooth muscle, neural tract, adipose tissue, and the cardiovascular system, where they are expressed in endothelial cells, vascular smooth muscle, cardiomyocytes, and different inflammatory cells [20], underpinning the synthetic GLP-1 and GLP-1RAs associated cardiovascular benefits observed in clinics [21].
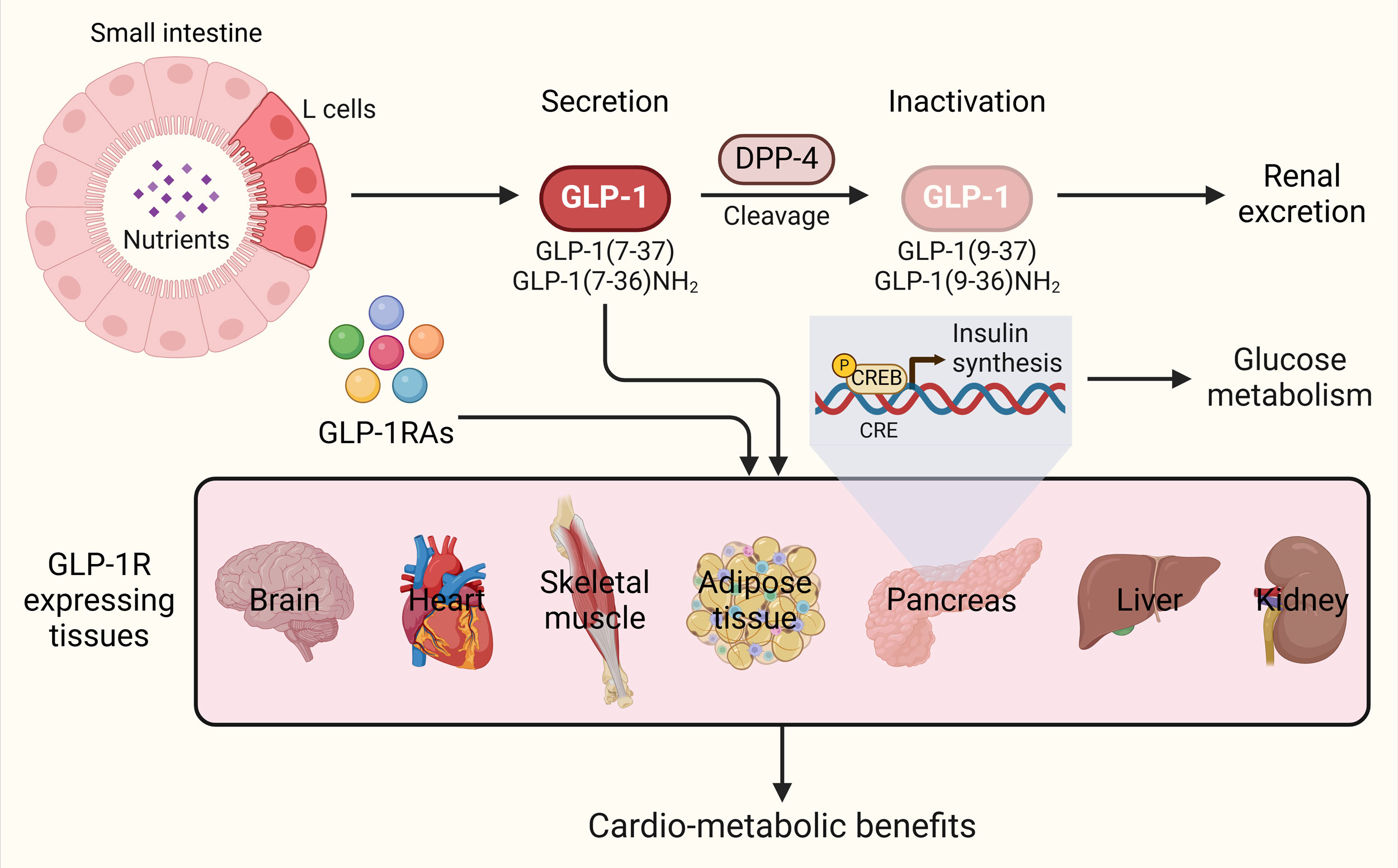
GLP-1RAs maintain vascular health
GLP-1 plays crucial role in proper functioning of vascular endothelium, and is linked to improved endothelial function, and enhanced nitric oxide synthase-driven vasodilation [22]. GLP-1RAs promote healthy vascular function by targeting the AMP-activated protein kinase (AMPK)/Sirtuin 1 (SIRT1)/FoxO3a pathway, maintaining a calponin+SM22α+ vascular smooth muscle cell phenotype [27]. Moreover, GLP-1RAs may also promote vasodilation even in conditions with where Nox-1/endothelin-1 are low [28]. GLP-1RAs have been shown to directly attenuate atherosclerotic progression. In animal models, GLP-1RA therapy has been shown to slows atherosclerosis by inhibiting angiotensin-II-induced proliferation of vascular smooth muscle cells via AMPK activation [29]. In addition, GLP-1RAs may stabilize plaques by enhancing SIRT6 levels [30]. Liraglutide and semaglutide treatment has been found to decrease aortic intimal thickening and plaque area in different atherosclerotic mice models [31]. Dulaglutide similarly reduced plaque area in Apoe−/− atherosclerotic mice, with early-stage diabetes intervention resulting in significantly lower infiltration of macrophages into aortic root lesions, compared to late-stage treatment [32]. Exenatide, has been shown to enhance the collagen amount in plaques while reducing matrix metalloproteinase activity, contributing to plaque stabilization [33]. This effect is believed to occur through the activation of tissue inhibitors of matrix metalloproteinases, natural inhibitors of MMPs [34]. Furthermore, semaglutide has been shown to reduce the CD163+ macrophages in atherosclerotic plaques [35]. Ongoing human studies aim to determine whether GLP-1RAs reduce plaque formation directly or they just stabilize the plaque avoiding its rupture [36].
GLP-1R agonism also mitigate atherosclerosis-driven lipotoxic stress imposed on the endoplasmic reticulum in endothelial cells and macrophages; hence preventing necrotic core formation that leads to plaque ruptures [37]. Liraglutide has demonstrated the ability to mitigate endoplasmic reticulum stress induced by dextrose and tunicamycin in human coronary artery endothelial cells. This is achieved through the suppression of glucose-regulated protein 78 and activating transcription factor 6 expression, as well as the inhibition of protein kinase RNA-like ER kinase and IRE1α phosphorylation. Notably, liraglutide exhibits greater effectiveness in this regard when compared to sodium-glucose cotransporter-2 (SGLT2) inhibitors or metformin [38]. In addition, exendin-4 activates p38, potentially protecting against lipoapoptosis [39]. GLP-1RAs have also shown anti-thrombotic effects. Exenatide has been shown to inhibit platelet aggregation triggered by thrombin, adenosine diphosphate, and collagen [40], while liraglutide has been shown to significantly reduce platelet aggregation induced by thromboxane in obese and prediabetic participants [41]. GLP-1RAs, including exenatide and liraglutide, have also been demonstrated to influence platelet biology by decreasing arachidonic acid-induced oxidative stress [42]. Additionally, GLP-1 receptor activation may inhibit platelet activation by increasing nitric oxide levels and decreasing P-selectin and platelet activation complex-1 expression [43]. Altogether, GLP-1RAs are potent modulators of vascular health, potentially mitigating atherogenesis, preventing plaque rupture, and reducing the risk of atherothrombotic events.
GLP-1RAs as anti-inflammatory agents
GLP-1R agonism is believed to exert atheroprotective effects by reducing inflammation both systemically and locally within the arterial wall [23]. GLP-1RAs appear to modulate macrophage biology. For instance, liraglutide promoted an increase in M2-like macrophages in Apoe−/− mice, and upregulated anti-inflammatory factors, including Arg-1 and IL-10, within aortic plaques [44]. On the other hand, in Apoe−/− Irs2+/− mice, lixisenatide decreased plaque size and the necrotic core area in aortic plaques while reducing M1-like pro-inflammatory macrophages and promoting M2-like anti-inflammatory macrophages [45]. In addition, GLP-1RAs prevents the formation of the NLRP3 inflammasome, thus safeguard from autoinflammatory damage induced by hypergylcemia, resulting in anti-pyroptotic beneficial effects to cardiomyocytes [46]. GLP-1 receptor activation can suppress the transcription of inflammation pathway-related genes like superoxide dismutase 2 and nuclear factor kappa-B [47]. Additionally, semaglutide has also been found to reduce plasma concentrations of interferon-γ and tumor necrosis factor-α (TNF-α), while simultaneously lowering mRNA expression levels of cytokines such as chemokine ligand 2, interleukin-6 and vascular cell adhesion molecule-1 [31]. On the other hand, liraglutide has demonstrated the ability to alleviate TNF-α-induced inflammation and reduce monocyte adhesion to the vascular endothelium by suppressing the expression of VCAM-1 and E-selectin. These effects are mediated through the upregulation of calcium/calmodulin-dependent protein kinase I and cAMP response element binding protein (CREB), along with the activation of the CaMKKβ/AMPK signaling pathways [48]. Additionally, GLP-1RAs activate the extracellular-signal-regulated kinase 5 pathway, which enhances the expression of Krüppel-like factor 2. This activation prevents the inhibition of mitogen-activated protein kinase activity and triggers anti-inflammatory mechanisms, including reduced leukocyte adhesion [49]. Together, these findings underscore the robust anti-inflammatory properties of GLP-1RAs, highlighting their therapeutic potential in managing atherosclerotic cardiovascular diseases.
GLP-1RAs counteract diabetic hyperglycemia and dyslipidemia
GLP-1RAs effectively treat hyperglycemia and decrease glycated hemoglobin (HbA1c) levels in individuals with T2DM. Among head-to-head comparisons, semaglutide and liraglutide have emerged as the most effective GLP-1RAs for lowering HbA1c levels [15]. On the other hand, GLP-1R agonism typically produce a modest but favorable effect on blood lipid profiles. At molecular level, GLP-1RAs significantly impact cholesterol regulation by facilitating the activation of ATP-binding cassette transporter A1 (ABCA1), resulting in removal of cholesterol from foam cells [50]. They also suppress the production of acetyl-CoA acetyltransferase 1 [51] and strengthen the signaling between the adaptor protein APPL1 and adiponectin, a mechanism that effectively reduces the development of foam cells [52]. Additionally, co-agonism of GLP-1/glucagon promotes reverse cholesterol transport by upregulating low density lipoprotein receptor/ABCA1 and cholesterol 7α-hydroxylase/ABCB11, respectively [53]. Oral semaglutide resulted in a 4–5% reduction in total cholesterol and LDL cholesterol (LDL-c), as well as a 12% decrease in triglycerides compared to baseline levels in the PIONEER 6 study [54]. Similarly, subcutaneous semaglutide showed a 7–8% reduction in triglycerides along with a 3% reduction in both total cholesterol and LDL-c [55]. Liraglutide may also contribute to a reduction in postprandial remnant cholesterol, potentially through lowering ApoC III levels [56]. Moreover, a comprehensive lipidomic analysis demonstrated that liraglutide significantly reduces various lipid species, such as triglycerides, ceramides, phosphatidylethanolamines and phosphatidylcholines [57]. Meta-analyses have also highlighted that GLP-1RAs are linked to modest reductions in total cholesterol, LDL-c, and triglycerides [58]. However, evidence of direct causal connection between this reduction of lipids and improved cardiovascular outcomes is still lacking.
GLP-1R agonism downregulates lipogenic genes and hyperactivates lipolysis in human adipocytes, thereby helping to mitigate a systemic obesogenic environment [25]. Dysregulation of adipokines significantly contributes to insulin resistance in T2DM. Specifically, low levels of adiponectin in serum serve as an independent biomarker for CAD severity and future atherosclerotic CVD events [59]. In this context, exendin-4 has demonstrated to elevate adiponectin levels through the Sirt1/FoxO1 signaling pathway [60]. Furthermore, a meta-analysis of 20 trials has indicated that liraglutide significantly upregulates circulating adiponectin levels, irrespective of changes in fat mass [61]. Combining GLP-1RAs with glucagon significantly inhibits fat production by suppressing the expression of sterol regulatory element-binding protein-1c and β-hydroxy β-methylglutaryl-CoA reductase. Dysfunctional epicardial adipose tissue contributes to a pro-inflammatory environment that impacts neighboring coronary arteries, thereby playing a significant role in the development of atherosclerotic CVD [62]. Epicardial adipose tissue expresses GLP-1R at levels significantly higher than in subcutaneous tissue [63], suggesting that GLP-1RAs may offer cardiovascular benefits by targeting epicardial adipose tissue. Both semaglutide and dulaglutide have shown dose-dependent reductions in EAT thickness in obese individuals with T2DM [64]. In summary, GLP-1RAs mediated management of hyperglycemia and dyslipidemia in T2DM exhibit potential cardiovascular benefits through multifaceted mechanisms, including glucose and lipid modulation, adiponectin regulation, and epicardial adipose tissue maintenance, underscoring their comprehensive therapeutic value.
GLP-1RAs as weight loss therapy
Reducing body weight has been shown to limit obesity-related CVD risk factors and alleviate inflammation, beneficial for long-term health outcomes [65, 66]. Additionally, weight loss is linked to improved cardiac prognosis, reducing the occurrence of adverse events such as urgent revascularizations, acute atherosclerotic CVD incidents, and overall mortality [26]. In particular, obesity-related diabetes has been associated with increased coronary plaque burden [67]. Among the pharmacological treatments available for obesity, GLP-1RAs are among the most potent, although their effectiveness varies depending on the specific agent. In the SUSTAIN-6 study, individuals who received subcutaneous semaglutide experienced average weight losses of 3.6 kg and 4.9 kg at doses of 0.5 mg and 1.0 mg, respectively. However, those who were given a placebo experienced reductions of 0.7 kg and 0.5 kg [55]. In the STEP1 trial, semaglutide (2.4 mg) induced a mean weight loss of 14.9% among overweight or obese patients who did not have T2DM [68].
Although interest in using GLP-1RAs for obesity treatment is notably growing, it is tempting to directly associate the cardiovascular improvements seen with GLP-1RA therapy to weight reduction as the extent of weight loss induced by GLP-1RAs does not completely explain the observed cardiovascular benefits [69]. Although the cardiovascular advantages of GLP-1RAs in diabetic patients are well-documented [8], there has been limited evidence to support their effectiveness in reducing CVD risk in individuals who are obese or overweight but do not have diabetes. Key evidence supporting the notion came from the SELECT trial, which included 17,604 participants aged 45 and older from over 800 locations, recently demonstrated that weekly subcutaneous injections of 2.4 mg of semaglutide significantly reduced MACEs by 20% compared to a placebo in overweight and obese individuals with existing atherosclerotic CVD but no diabetes [26]. Additionally, post hoc analysis revealed that weight loss in this group was maintained for up to four years [70]. Notably, the cardiovascular benefits of semaglutide began to emerge within three months of treatment, before significant weight loss or other cardiometabolic improvements were observed, suggesting that mechanisms beyond weight reduction contribute to the drug's cardioprotective effects [26]. First approved by FDA in 2021, semaglutide 2.4 mg (Wegovy) was intended for long-term weight management in adults diagnosed with obesity or those who are overweight and have at least one health condition related to their weight. Recently, it has received expanded FDA approval. The new indication includes reducing cardiovascular risk in individuals with both cardiovascular disease and obesity/overweight.
Based on the fact that atherosclerosis affects noncoronary areas as well, the impact of GLP-1RAs on peripheral arterial vasculature becomes particularly significant. However, compared to their effects on CAD and stroke, there is relatively limited data regarding the impact of GLP-1RAs on PAD outcomes. Most of the major outcome trials were underpowered to assess these events, as patients with CAD outnumbered those with PAD in these studies. For instance, PAD was represented in only 12.7% of participants in LEADER and 14.0% in SUSTAIN-6 trial [75]. Additionally, the ELIXA trial did not include PAD in its eligibility criteria [76]. Moreover, data on revascularization outcomes were often aggregated, combining coronary and peripheral interventions, which hindered targeted analysis of PAD-related events. Therefore, further studies with cohorts enriched for PAD patients and prespecified PAD outcomes are necessary. Despite the limited data, existing evidence suggests that GLP-1RAs are safe and may contribute to a reduction in PAD-related events [77]. A cohort study conducted in Denmark with 309,166 individuals diagnosed with diabetes revealed that treatment with GLP-1RAs led to a 50% decrease in amputations related to diabetes, when compared to those who did not receive GLP-1RA treatment [78]. These findings, along with indications of safety and potential benefits for PAD outcomes, suggest that GLP-1RAs could be considered a preferred therapy over other anti-diabetic medications for patients with concurrent T2DM and PAD [79]. This is particularly important in light of the findings from the CANVAS program, which revealed that the SGLT2 inhibitor canagliflozin was associated with a doubled risk of lower limb amputation when compared to a placebo [80]. Additionally, a recent meta-analysis reinforced the advantages of GLP-1RAs, demonstrating a significantly reduced occurrence of lower limb amputations in comparison to SGLT2 inhibitors [81]. Several ongoing studies aim to further explore how GLP-1RAs may influence peripheral endothelial function in patients with PAD. The SAMAS trial (NCT05147896) is also underway, aiming to examine the impact of oral semaglutide on various markers of atherosclerotic cardiovascular disease, including arterial stiffness, carotid intima-media thickness, and endothelial function. Additionally, the trial will evaluate its effects on atherosclerotic risk factors, such as LDL-c, HbA1c levels, and high-sensitivity C-reactive protein [82].
GLP-1RAs have been linked to significant reductions in cerebrovascular incidents. Clinical trials, such as REWIND with dulaglutide and SUSTAIN-6 with subcutaneous semaglutide, reported relative decreases in nonfatal stroke rates by 24% and 39%, respectively, when compared to placebo groups [83]. However, these positive outcomes were not consistently observed across all GLP-1RA studies. Meta-analyses indicate that the improvement in stroke outcomes is largely attributed to reductions in ischemic stroke rather than hemorrhagic stroke [84]. For primary prevention, GLP-1RAs have demonstrated approximately a 16% decrease in the risk of both nonfatal and total strokes [85]. Among diabetes treatments, GLP-1RAs and thiazolidinediones stand out as the only two medication classes shown to effectively lower stroke risk [86]. In conclusion, GLP-1RAs offer substantial cardiovascular benefits across a spectrum of cardiovascular conditions at clinical level, highlighting their pivotal role in the management of CVDs while underscoring the need for further research to optimize their clinical application.
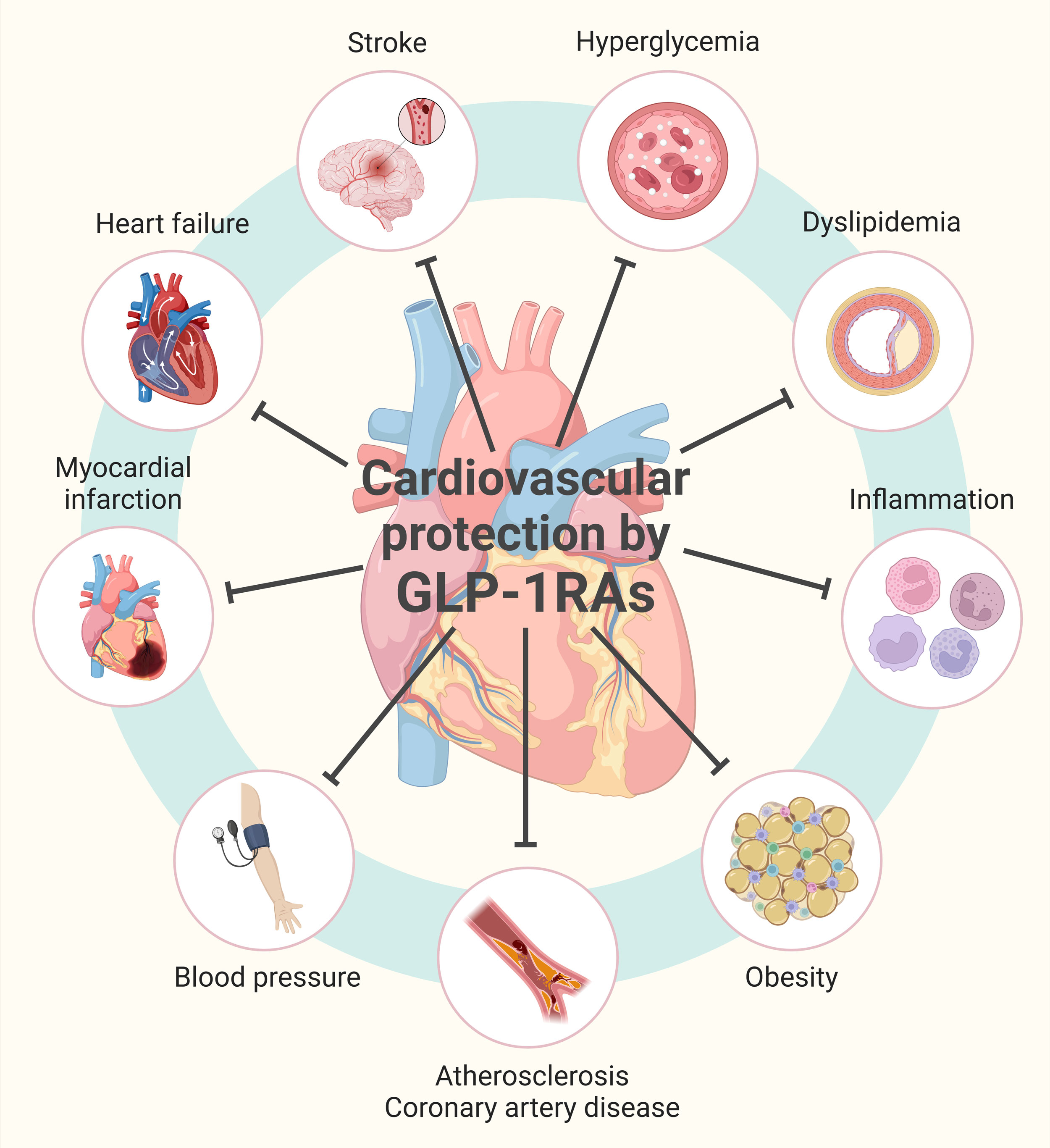
No applicable.
Ethics approval
No applicable.
Data availability
The data will be available upon request.
Funding
None.
Authors’ contribution
HS wrote the manuscript draft, devoted to to the figure production, and submitted the final manuscript.
Competing interests
I declare that there are no conflicts of interest regarding the publication of this document. I confirm that neither I nor any of my collaborators have any financial or personal relationships that could inappropriately influence or bias the content of this study.
- Roth GA, Mensah GA, Johnson CO, Addolorato G, Ammirati E, Baddour LM, Barengo NC, Beaton AZ, Benjamin EJ, Benziger CP, et al: Global Burden of Cardiovascular Diseases and Risk Factors, 1990-2019: Update From the GBD 2019 Study. J Am Coll Cardiol 2020, 76(25): 2982-3021.
- Libby P: The changing landscape of atherosclerosis. Nature 2021, 592(7855): 524-533.
- Wolf D, Ley K: Immunity and Inflammation in Atherosclerosis. Circ Res 2019, 124(2): 315-327.
- Saeedi P, Petersohn I, Salpea P, Malanda B, Karuranga S, Unwin N, Colagiuri S, Guariguata L, Motala AA, Ogurtsova K, et al: Global and regional diabetes prevalence estimates for 2019 and projections for 2030 and 2045: Results from the International Diabetes Federation Diabetes Atlas, 9(th) edition. Diabetes Res Clin Pract 2019, 157: 107843.
- Bhupathiraju SN, Hu FB: Epidemiology of Obesity and Diabetes and Their Cardiovascular Complications. Circ Res 2016, 118(11): 1723-1735.
- Hwang TJ, Franklin JM, Kesselheim AS: Effect of US Food and Drug Administration's Cardiovascular Safety Guidance on Diabetes Drug Development. Clin Pharmacol Ther 2017, 102(2): 290-296.
- Mariam Z, Niazi SK: Glucagon-like peptide agonists: A prospective review. Endocrinol Diabetes Metab 2024, 7(1): e462.
- Sattar N, Lee MMY, Kristensen SL, Branch KRH, Del Prato S, Khurmi NS, Lam CSP, Lopes RD, McMurray JJV, Pratley RE, et al: Cardiovascular, mortality, and kidney outcomes with GLP-1 receptor agonists in patients with type 2 diabetes: a systematic review and meta-analysis of randomised trials. Lancet Diabetes Endocrinol 2021, 9(10): 653-662.
- Honigberg MC, Chang LS, McGuire DK, Plutzky J, Aroda VR, Vaduganathan M: Use of Glucagon-Like Peptide-1 Receptor Agonists in Patients With Type 2 Diabetes and Cardiovascular Disease: A Review. JAMA Cardiol 2020, 5(10): 1182-1190.
- Holst JJ, Gromada J: Role of incretin hormones in the regulation of insulin secretion in diabetic and nondiabetic humans. Am J Physiol Endocrinol Metab 2004, 287(2): E199-206.
- Müller TD, Finan B, Bloom SR, D'Alessio D, Drucker DJ, Flatt PR, Fritsche A, Gribble F, Grill HJ, Habener JF, et al: Glucagon-like peptide 1 (GLP-1). Mol Metab 2019, 30: 72-130.
- Nauck MA, Meier JJ: Incretin hormones: Their role in health and disease. Diabetes Obes Metab 2018, 20 Suppl 1: 5-21.
- Nauck MA, Heimesaat MM, Behle K, Holst JJ, Nauck MS, Ritzel R, Hüfner M, Schmiegel WH: Effects of glucagon-like peptide 1 on counterregulatory hormone responses, cognitive functions, and insulin secretion during hyperinsulinemic, stepped hypoglycemic clamp experiments in healthy volunteers. J Clin Endocrinol Metab 2002, 87(3): 1239-1246.
- Le R, Nguyen MT, Allahwala MA, Psaltis JP, Marathe CS, Marathe JA, Psaltis PJ: Cardiovascular Protective Properties of GLP-1 Receptor Agonists: More than Just Diabetic and Weight Loss Drugs. J Clin Med 2024, 13(16): 4674.
- Leech CA, Chepurny OG, Holz GG: Epac2-dependent rap1 activation and the control of islet insulin secretion by glucagon-like peptide-1. Vitam Horm 2010, 84: 279-302.
- Pang B, Kim S, Li D, Ma Z, Sun B, Zhang X, Wu Z, Chen L: Glucagon-like peptide-1 potentiates glucose-stimulated insulin secretion via the transient receptor potential melastatin 2 channel. Exp Ther Med 2017, 14(5): 5219-5227.
- Jones B, McGlone ER, Fang Z, Pickford P, Corrêa IR, Jr., Oishi A, Jockers R, Inoue A, Kumar S, Görlitz F, et al: Genetic and biased agonist-mediated reductions in β-arrestin recruitment prolong cAMP signaling at glucagon family receptors. J Biol Chem 2021, 296: 100133.
- Miao XY, Gu ZY, Liu P, Hu Y, Li L, Gong YP, Shu H, Liu Y, Li CL: The human glucagon-like peptide-1 analogue liraglutide regulates pancreatic beta-cell proliferation and apoptosis via an AMPK/mTOR/P70S6K signaling pathway. Peptides 2013, 39: 71-79.
- Carlessi R, Chen Y, Rowlands J, Cruzat VF, Keane KN, Egan L, Mamotte C, Stokes R, Gunton JE, Bittencourt PIH, et al: GLP-1 receptor signalling promotes β-cell glucose metabolism via mTOR-dependent HIF-1α activation. Sci Rep 2017, 7(1): 2661.
- Drucker DJ: Mechanisms of Action and Therapeutic Application of Glucagon-like Peptide-1. Cell Metab 2018, 27(4): 740-756.
- Helmstädter J, Keppeler K, Küster L, Münzel T, Daiber A, Steven S: Glucagon-like peptide-1 (GLP-1) receptor agonists and their cardiovascular benefits-The role of the GLP-1 receptor. Br J Pharmacol 2022, 179(4): 659-676.
- Ban K, Noyan-Ashraf MH, Hoefer J, Bolz SS, Drucker DJ, Husain M: Cardioprotective and vasodilatory actions of glucagon-like peptide 1 receptor are mediated through both glucagon-like peptide 1 receptor-dependent and -independent pathways. Circulation 2008, 117(18): 2340-2350.
- Nguyen MT, Fernando S, Schwarz N, Tan JT, Bursill CA, Psaltis PJ: Inflammation as a Therapeutic Target in Atherosclerosis. J Clin Med 2019, 8(8): 1109.
- Xia L, Shen T, Dong W, Su F, Wang J, Wang Q, Niu S, Fang Y: Comparative efficacy and safety of 8 GLP-1RAs in patients with type 2 diabetes: A network meta-analysis. Diabetes Res Clin Pract 2021, 177: 108904.
- El Bekay R, Coín-Aragüez L, Fernández-García D, Oliva-Olivera W, Bernal-López R, Clemente-Postigo M, Delgado-Lista J, Diaz-Ruiz A, Guzman-Ruiz R, Vázquez-Martínez R, et al: Effects of glucagon-like peptide-1 on the differentiation and metabolism of human adipocytes. Br J Pharmacol 2016, 173(11): 1820-1834.
- Lincoff AM, Brown-Frandsen K, Colhoun HM, Deanfield J, Emerson SS, Esbjerg S, Hardt-Lindberg S, Hovingh GK, Kahn SE, Kushner RF, et al: Semaglutide and Cardiovascular Outcomes in Obesity without Diabetes. N Engl J Med 2023, 389(24): 2221-2232.
- Liu Z, Zhang M, Zhou T, Shen Q, Qin X: Exendin-4 promotes the vascular smooth muscle cell re-differentiation through AMPK/SIRT1/FOXO3a signaling pathways. Atherosclerosis 2018, 276: 58-66.
- Sukumaran V, Tsuchimochi H, Sonobe T, Waddingham MT, Shirai M, Pearson JT: Liraglutide treatment improves the coronary microcirculation in insulin resistant Zucker obese rats on a high salt diet. Cardiovasc Diabetol 2020, 19(1): 24.
- Jojima T, Uchida K, Akimoto K, Tomotsune T, Yanagi K, Iijima T, Suzuki K, Kasai K, Aso Y: Liraglutide, a GLP-1 receptor agonist, inhibits vascular smooth muscle cell proliferation by enhancing AMP-activated protein kinase and cell cycle regulation, and delays atherosclerosis in ApoE deficient mice. Atherosclerosis 2017, 261: 44-51.
- Balestrieri ML, Rizzo MR, Barbieri M, Paolisso P, D'Onofrio N, Giovane A, Siniscalchi M, Minicucci F, Sardu C, D'Andrea D, et al: Sirtuin 6 expression and inflammatory activity in diabetic atherosclerotic plaques: effects of incretin treatment. Diabetes 2015, 64(4): 1395-1406.
- Rakipovski G, Rolin B, Nøhr J, Klewe I, Frederiksen KS, Augustin R, Hecksher-Sørensen J, Ingvorsen C, Polex-Wolf J, Knudsen LB: The GLP-1 Analogs Liraglutide and Semaglutide Reduce Atherosclerosis in ApoE(-/-) and LDLr(-/-) Mice by a Mechanism That Includes Inflammatory Pathways. JACC Basic Transl Sci 2018, 3(6): 844-857.
- Sanada J, Obata A, Obata Y, Fushimi Y, Shimoda M, Kohara K, Nakanishi S, Mune T, Kaku K, Kaneto H: Dulaglutide exerts beneficial anti atherosclerotic effects in ApoE knockout mice with diabetes: the earlier, the better. Sci Rep 2021, 11(1): 1425.
- Yang G, Lei Y, Inoue A, Piao L, Hu L, Jiang H, Sasaki T, Wu H, Xu W, Yu C, et al: Exenatide mitigated diet-induced vascular aging and atherosclerotic plaque growth in ApoE-deficient mice under chronic stress. Atherosclerosis 2017, 264: 1-10.
- Garczorz W, Gallego-Colon E, Kosowska A, Kłych-Ratuszny A, Woźniak M, Marcol W, Niesner KJ, Francuz T: Exenatide exhibits anti-inflammatory properties and modulates endothelial response to tumor necrosis factor α-mediated activation. Cardiovasc Ther 2018, 36(2): e12317.
- Guo L, Akahori H, Harari E, Smith SL, Polavarapu R, Karmali V, Otsuka F, Gannon RL, Braumann RE, Dickinson MH, et al: CD163+ macrophages promote angiogenesis and vascular permeability accompanied by inflammation in atherosclerosis. J Clin Invest 2018, 128(3): 1106-1124.
- Hamal S, Cherukuri L, Shaikh K, Kinninger A, Doshi J, Birudaraju D, Budoff MJ: Effect of semaglutide on coronary atherosclerosis progression in patients with type II diabetes: rationale and design of the semaglutide treatment on coronary progression trial. Coron Artery Dis 2020, 31(3): 306-314.
- Ni L, Yang L, Lin Y: Recent progress of endoplasmic reticulum stress in the mechanism of atherosclerosis. Front Cardiovasc Med 2024, 11: 1413441.
- Kapadia P, Bikkina P, Landicho MA, Parekh S, Haas MJ, Mooradian AD: Effect of anti-hyperglycemic drugs on endoplasmic reticulum (ER) stress in human coronary artery endothelial cells. Eur J Pharmacol 2021, 907: 174249.
- Erdogdu O, Eriksson L, Xu H, Sjöholm A, Zhang Q, Nyström T: Exendin-4 protects endothelial cells from lipoapoptosis by PKA, PI3K, eNOS, p38 MAPK, and JNK pathways. J Mol Endocrinol 2013, 50(2): 229-241.
- Cameron-Vendrig A, Reheman A, Siraj MA, Xu XR, Wang Y, Lei X, Afroze T, Shikatani E, El-Mounayri O, Noyan H, et al: Glucagon-Like Peptide 1 Receptor Activation Attenuates Platelet Aggregation and Thrombosis. Diabetes 2016, 65(6): 1714-1723.
- Cahill KN, Amin T, Boutaud O, Printz R, Newcomb DC, Foer D, Hodson DJ, Broichhagen J, Beckman JA, Yu C, et al: Glucagon-Like Peptide-1 Receptor Regulates Thromboxane-Induced Human Platelet Activation. JACC Basic Transl Sci 2022, 7(7): 713-715.
- Barale C, Buracco S, Cavalot F, Frascaroli C, Guerrasio A, Russo I: Glucagon-like peptide 1-related peptides increase nitric oxide effects to reduce platelet activation. Thromb Haemost 2017, 117(6): 1115-1128.
- Zhang Y, Chen R, Jia Y, Chen M, Shuai Z: Effects of Exenatide on Coagulation and Platelet Aggregation in Patients with Type 2 Diabetes. Drug Des Devel Ther 2021, 15: 3027-3040.
- Bruen R, Curley S, Kajani S, Crean D, O'Reilly ME, Lucitt MB, Godson CG, McGillicuddy FC, Belton O: Liraglutide dictates macrophage phenotype in apolipoprotein E null mice during early atherosclerosis. Cardiovasc Diabetol 2017, 16(1): 143.
- Vinué Á, Navarro J, Herrero-Cervera A, García-Cubas M, Andrés-Blasco I, Martínez-Hervás S, Real JT, Ascaso JF, González-Navarro H: The GLP-1 analogue lixisenatide decreases atherosclerosis in insulin-resistant mice by modulating macrophage phenotype. Diabetologia 2017, 60(9): 1801-1812.
- Wei H, Bu R, Yang Q, Jia J, Li T, Wang Q, Chen Y: Exendin-4 Protects against Hyperglycemia-Induced Cardiomyocyte Pyroptosis via the AMPK-TXNIP Pathway. J Diabetes Res 2019, 2019: 8905917.
- Scisciola L, Rizzo MR, Cataldo V, Fontanella RA, Balestrieri ML, D'Onofrio N, Marfella R, Paolisso G, Barbieri M: Incretin drugs effect on epigenetic machinery: New potential therapeutic implications in preventing vascular diabetic complications. Faseb j 2020, 34(12): 16489-16503.
- Krasner NM, Ido Y, Ruderman NB, Cacicedo JM: Glucagon-like peptide-1 (GLP-1) analog liraglutide inhibits endothelial cell inflammation through a calcium and AMPK dependent mechanism. PLoS One 2014, 9(5): e97554.
- Yue W, Li Y, Ou D, Yang Q: The GLP-1 receptor agonist liraglutide protects against oxidized LDL-induced endothelial inflammation and dysfunction via KLF2. IUBMB Life 2019, 71(9): 1347-1354.
- Hu YW, Yang JY, Ma X, Chen ZP, Hu YR, Zhao JY, Li SF, Qiu YR, Lu JB, Wang YC, et al: A lincRNA-DYNLRB2-2/GPR119/GLP-1R/ABCA1-dependent signal transduction pathway is essential for the regulation of cholesterol homeostasis. J Lipid Res 2014, 55(4): 681-697.
- Tashiro Y, Sato K, Watanabe T, Nohtomi K, Terasaki M, Nagashima M, Hirano T: A glucagon-like peptide-1 analog liraglutide suppresses macrophage foam cell formation and atherosclerosis. Peptides 2014, 54: 19-26.
- Barbieri M, Marfella R, Esposito A, Rizzo MR, Angellotti E, Mauro C, Siniscalchi M, Chirico F, Caiazzo P, Furbatto F, et al: Incretin treatment and atherosclerotic plaque stability: Role of adiponectin/APPL1 signaling pathway. J Diabetes Complications 2017, 31(2): 295-303.
- Patel V, Joharapurkar A, Kshirsagar S, Sutariya B, Patel M, Pandey D, Patel H, Ranvir R, Kadam S, Patel D, et al: Coagonist of GLP-1 and glucagon decreases liver inflammation and atherosclerosis in dyslipidemic condition. Chem Biol Interact 2018, 282: 13-21.
- Husain M, Birkenfeld AL, Donsmark M, Dungan K, Eliaschewitz FG, Franco DR, Jeppesen OK, Lingvay I, Mosenzon O, Pedersen SD, et al: Oral Semaglutide and Cardiovascular Outcomes in Patients with Type 2 Diabetes. N Engl J Med 2019, 381(9): 841-851.
- Marso SP, Bain SC, Consoli A, Eliaschewitz FG, Jódar E, Leiter LA, Lingvay I, Rosenstock J, Seufert J, Warren ML, et al: Semaglutide and Cardiovascular Outcomes in Patients with Type 2 Diabetes. N Engl J Med 2016, 375(19): 1834-1844.
- Matikainen N, Söderlund S, Björnson E, Pietiläinen K, Hakkarainen A, Lundbom N, Taskinen MR, Borén J: Liraglutide treatment improves postprandial lipid metabolism and cardiometabolic risk factors in humans with adequately controlled type 2 diabetes: A single-centre randomized controlled study. Diabetes Obes Metab 2019, 21(1): 84-94.
- Zobel EH, Wretlind A, Ripa RS, Rotbain Curovic V, von Scholten BJ, Suvitaival T, Hansen TW, Kjær A, Legido-Quigley C, Rossing P: Ceramides and phospholipids are downregulated with liraglutide treatment: results from the LiraFlame randomized controlled trial. BMJ Open Diabetes Res Care 2021, 9(1): e002395.
- Sun F, Wu S, Wang J, Guo S, Chai S, Yang Z, Li L, Zhang Y, Ji L, Zhan S: Effect of glucagon-like peptide-1 receptor agonists on lipid profiles among type 2 diabetes: a systematic review and network meta-analysis. Clin Ther 2015, 37(1): 225-241.e228.
- Zhao S, Kusminski CM, Scherer PE: Adiponectin, Leptin and Cardiovascular Disorders. Circ Res 2021, 128(1): 136-149.
- Wang A, Li T, An P, Yan W, Zheng H, Wang B, Mu Y: Exendin-4 Upregulates Adiponectin Level in Adipocytes via Sirt1/Foxo-1 Signaling Pathway. PLoS One 2017, 12(1): e0169469.
- Simental-Mendía LE, Sánchez-García A, Linden-Torres E, Simental-Mendía M: Impact of glucagon-like peptide-1 receptor agonists on adiponectin concentrations: A meta-analysis of randomized controlled trials. Br J Clin Pharmacol 2021, 87(11): 4140-4149.
- McAninch EA, Fonseca TL, Poggioli R, Panos AL, Salerno TA, Deng Y, Li Y, Bianco AC, Iacobellis G: Epicardial adipose tissue has a unique transcriptome modified in severe coronary artery disease. Obesity (Silver Spring) 2015, 23(6): 1267-1278.
- Iacobellis G, Camarena V, Sant DW, Wang G: Human Epicardial Fat Expresses Glucagon-Like Peptide 1 and 2 Receptors Genes. Horm Metab Res 2017, 49(8): 625-630.
- Iacobellis G, Villasante Fricke AC: Effects of Semaglutide Versus Dulaglutide on Epicardial Fat Thickness in Subjects with Type 2 Diabetes and Obesity. J Endocr Soc 2020, 4(4): bvz042.
- Wing RR, Lang W, Wadden TA, Safford M, Knowler WC, Bertoni AG, Hill JO, Brancati FL, Peters A, Wagenknecht L: Benefits of modest weight loss in improving cardiovascular risk factors in overweight and obese individuals with type 2 diabetes. Diabetes Care 2011, 34(7): 1481-1486.
- Fuster JJ, Ouchi N, Gokce N, Walsh K: Obesity-Induced Changes in Adipose Tissue Microenvironment and Their Impact on Cardiovascular Disease. Circ Res 2016, 118(11): 1786-1807.
- Kwan AC, May HT, Cater G, Sibley CT, Rosen BD, Lima JA, Rodriguez K, Lappe DL, Muhlestein JB, Anderson JL, et al: Coronary artery plaque volume and obesity in patients with diabetes: the factor-64 study. Radiology 2014, 272(3): 690-699.
- Wilding JPH, Batterham RL, Calanna S, Davies M, Van Gaal LF, Lingvay I, McGowan BM, Rosenstock J, Tran MTD, Wadden TA, et al: Once-Weekly Semaglutide in Adults with Overweight or Obesity. N Engl J Med 2021, 384(11): 989-1002.
- Ghusn W, De la Rosa A, Sacoto D, Cifuentes L, Campos A, Feris F, Hurtado MD, Acosta A: Weight Loss Outcomes Associated With Semaglutide Treatment for Patients With Overweight or Obesity. JAMA Netw Open 2022, 5(9): e2231982.
- Ryan DH, Lingvay I, Deanfield J, Kahn SE, Barros E, Burguera B, Colhoun HM, Cercato C, Dicker D, Horn DB, et al: Long-term weight loss effects of semaglutide in obesity without diabetes in the SELECT trial. Nat Med 2024, 30(7): 2049-2057.
- Marso SP, Daniels GH, Brown-Frandsen K, Kristensen P, Mann JF, Nauck MA, Nissen SE, Pocock S, Poulter NR, Ravn LS, et al: Liraglutide and Cardiovascular Outcomes in Type 2 Diabetes. N Engl J Med 2016, 375(4): 311-322.
- Hernandez AF, Green JB, Janmohamed S, D'Agostino RB, Sr., Granger CB, Jones NP, Leiter LA, Rosenberg AE, Sigmon KN, Somerville MC, et al: Albiglutide and cardiovascular outcomes in patients with type 2 diabetes and cardiovascular disease (Harmony Outcomes): a double-blind, randomised placebo-controlled trial. Lancet 2018, 392(10157): 1519-1529.
- Gerstein HC, Sattar N, Rosenstock J, Ramasundarahettige C, Pratley R, Lopes RD, Lam CSP, Khurmi NS, Heenan L, Del Prato S, et al: Cardiovascular and Renal Outcomes with Efpeglenatide in Type 2 Diabetes. N Engl J Med 2021, 385(10): 896-907.
- Kosiborod MN, Abildstrøm SZ, Borlaug BA, Butler J, Rasmussen S, Davies M, Hovingh GK, Kitzman DW, Lindegaard ML, Møller DV, et al: Semaglutide in Patients with Heart Failure with Preserved Ejection Fraction and Obesity. N Engl J Med 2023, 389(12): 1069-1084.
- Verma S, Al-Omran M, Leiter LA, Mazer CD, Rasmussen S, Saevereid HA, Sejersten Ripa M, Bonaca MP: Cardiovascular efficacy of liraglutide and semaglutide in individuals with diabetes and peripheral artery disease. Diabetes Obes Metab 2022, 24(7): 1288-1299.
- Pfeffer MA, Claggett B, Diaz R, Dickstein K, Gerstein HC, Køber LV, Lawson FC, Ping L, Wei X, Lewis EF, et al: Lixisenatide in Patients with Type 2 Diabetes and Acute Coronary Syndrome. N Engl J Med 2015, 373(23): 2247-2257.
- Piechocki M, Przewłocki T, Pieniążek P, Trystuła M, Podolec J, Kabłak-Ziembicka A: A Non-Coronary, Peripheral Arterial Atherosclerotic Disease (Carotid, Renal, Lower Limb) in Elderly Patients-A Review: Part I-Epidemiology, Risk Factors, and Atherosclerosis-Related Diversities in Elderly Patients. J Clin Med 2024, 13(5): 1471.
- Schäfer Z, Mathisen A, Thomsen TR, Rossing P, Kirketerp-Møller K: Glucagon-like peptide-1 treatment reduces the risk of diabetes-type 2 related amputations: A cohort study in Denmark. Diabetes Res Clin Pract 2023, 202: 110799.
- Liarakos AL, Tentolouris A, Kokkinos A, Eleftheriadou I, Tentolouris N: Impact of Glucagon-like peptide 1 receptor agonists on peripheral arterial disease in people with diabetes mellitus: A narrative review. J Diabetes Complications 2023, 37(2): 108390.
- Neal B, Perkovic V, Mahaffey KW, de Zeeuw D, Fulcher G, Erondu N, Shaw W, Law G, Desai M, Matthews DR: Canagliflozin and Cardiovascular and Renal Events in Type 2 Diabetes. N Engl J Med 2017, 377(7): 644-657.
- Scheen AJ: Lower limb amputations: protection with GLP-1 receptor agonists rather than increased risk with SGLT2 inhibitors? Diabetes Metab 2022, 48(2): 101325.
- Janić M, Rizzo M, Cosentino F, Pantea Stoian A, Lunder M, Šabović M, Janež A: Effect of Oral Semaglutide on Cardiovascular Parameters and Their Mechanisms in Patients with Type 2 Diabetes: Rationale and Design of the Semaglutide Anti-Atherosclerotic Mechanisms of Action Study (SAMAS). Diabetes Ther 2022, 13(4): 795-810.
- Gerstein HC, Colhoun HM, Dagenais GR, Diaz R, Lakshmanan M, Pais P, Probstfield J, Riesmeyer JS, Riddle MC, Rydén L, et al: Dulaglutide and cardiovascular outcomes in type 2 diabetes (REWIND): a double-blind, randomised placebo-controlled trial. Lancet 2019, 394(10193): 121-130.
- Wei J, Yang B, Wang R, Ye H, Wang Y, Wang L, Zhang X: Risk of stroke and retinopathy during GLP-1 receptor agonist cardiovascular outcome trials: An eight RCTs meta-analysis. Front Endocrinol (Lausanne) 2022, 13: 1007980.
- Malhotra K, Katsanos AH, Lambadiari V, Goyal N, Palaiodimou L, Kosmidou M, Krogias C, Alexandrov AV, Tsivgoulis G: GLP-1 receptor agonists in diabetes for stroke prevention: a systematic review and meta-analysis. J Neurol 2020, 267(7): 2117-2122.
- Benn M, Emanuelsson F, Tybjærg-Hansen A, Nordestgaard BG: Impact of high glucose levels and glucose lowering on risk of ischaemic stroke: a Mendelian randomisation study and meta-analysis. Diabetologia 2021, 64(7): 1492-1503.
- Holman RR, Bethel MA, Mentz RJ, Thompson VP, Lokhnygina Y, Buse JB, Chan JC, Choi J, Gustavson SM, Iqbal N, et al: Effects of Once-Weekly Exenatide on Cardiovascular Outcomes in Type 2 Diabetes. N Engl J Med 2017, 377(13): 1228-1239.
- Mannucci E, Silverii GA: Cardiovascular prevention with glucose-lowering drugs in type 2 diabetes: An evidence-based approach to the categories of primary and secondary prevention. Diabetes Obes Metab 2023, 25(12): 3435-3443.
- Tsapas A, Avgerinos I, Karagiannis T, Malandris K, Manolopoulos A, Andreadis P, Liakos A, Matthews DR, Bekiari E: Comparative Effectiveness of Glucose-Lowering Drugs for Type 2 Diabetes: A Systematic Review and Network Meta-analysis. Ann Intern Med 2020, 173(4): 278-286.
- Sabatine MS, Giugliano RP, Keech AC, Honarpour N, Wiviott SD, Murphy SA, Kuder JF, Wang H, Liu T, Wasserman SM, et al: Evolocumab and Clinical Outcomes in Patients with Cardiovascular Disease. N Engl J Med 2017, 376(18): 1713-1722.
- Tardif JC, Kouz S, Waters DD, Bertrand OF, Diaz R, Maggioni AP, Pinto FJ, Ibrahim R, Gamra H, Kiwan GS, et al: Efficacy and Safety of Low-Dose Colchicine after Myocardial Infarction. N Engl J Med 2019, 381(26): 2497-2505.
- Svanström H, Ueda P, Melbye M, Eliasson B, Svensson AM, Franzén S, Gudbjörnsdottir S, Hveem K, Jonasson C, Pasternak B: Use of liraglutide and risk of major cardiovascular events: a register-based cohort study in Denmark and Sweden. Lancet Diabetes Endocrinol 2019, 7(2): 106-114.
- Trevisan M, Fu EL, Szummer K, Norhammar A, Lundman P, Wanner C, Sjölander A, Jernberg T, Carrero JJ: Glucagon-like peptide-1 receptor agonists and the risk of cardiovascular events in diabetes patients surviving an acute myocardial infarction. Eur Heart J Cardiovasc Pharmacother 2021, 7(2): 104-111.
- Cosentino F, Grant PJ, Aboyans V, Bailey CJ, Ceriello A, Delgado V, Federici M, Filippatos G, Grobbee DE, Hansen TB, et al: 2019 ESC Guidelines on diabetes, pre-diabetes, and cardiovascular diseases developed in collaboration with the EASD. Eur Heart J 2020, 41(2): 255-323.
- Buse JB, Wexler DJ, Tsapas A, Rossing P, Mingrone G, Mathieu C, D'Alessio DA, Davies MJ: 2019 Update to: Management of Hyperglycemia in Type 2 Diabetes, 2018. A Consensus Report by the American Diabetes Association (ADA) and the European Association for the Study of Diabetes (EASD). Diabetes Care 2020, 43(2): 487-493.
- Anholm C, Kumarathurai P, Pedersen LR, Samkani A, Walzem RL, Nielsen OW, Kristiansen OP, Fenger M, Madsbad S, Sajadieh A, et al: Liraglutide in combination with metformin may improve the atherogenic lipid profile and decrease C-reactive protein level in statin treated obese patients with coronary artery disease and newly diagnosed type 2 diabetes: A randomized trial. Atherosclerosis 2019, 288: 60-66.
- Wilding JPH, Batterham RL, Davies M, Van Gaal LF, Kandler K, Konakli K, Lingvay I, McGowan BM, Oral TK, Rosenstock J, et al: Weight regain and cardiometabolic effects after withdrawal of semaglutide: The STEP 1 trial extension. Diabetes Obes Metab 2022, 24(8): 1553-1564.
- Castellana M, Cignarelli A, Brescia F, Perrini S, Natalicchio A, Laviola L, Giorgino F: Efficacy and safety of GLP-1 receptor agonists as add-on to SGLT2 inhibitors in type 2 diabetes mellitus: A meta-analysis. Sci Rep 2019, 9(1): 19351.
- Chodorge M, Celeste AJ, Grimsby J, Konkar A, Davidsson P, Fairman D, Jenkinson L, Naylor J, White N, Seaman JC, et al: Engineering of a GLP-1 analogue peptide/anti-PCSK9 antibody fusion for type 2 diabetes treatment. Sci Rep 2018, 8(1): 17545.
Asia-Pacific Journal of Pharmacotherapy & Toxicology
p-ISSN: 2788-6840
e-ISSN: 2788-6859
Copyright © Asia Pac J Pharmacother Toxicol. This work is licensed under a Creative Commons Attribution-NonCommercial-No Derivatives 4.0 International (CC BY-NC-ND 4.0) License.